Home » c-Kit – The Novel Receptor: Physiological Roles, Downstream Signaling and Implications in Cancer
Research Article | Vol. 2, Issue 3 | Journal of Clinical Medical Research | Open Access |
c-Kit – The Novel Receptor: Physiological Roles, Downstream Signaling and Implications in Cancer
Bathri N Vajravelu1*, Tareq Al-Maqtari2
1Department of Physician Assistant Studies, Massachusetts College of Pharmacy and Health Sciences, Boston, MA, USA
2Department of Pharmacology, Sana’a University, Yemen
*Corresponding Author: Bathri N Vajravelu, PhD, MBBS, MPH, Department of Physician Assistant Studies Massachusetts College of Pharmacy and Health Sciences, Boston, MA, USA;
Email: [email protected]
Citation: Vajravelu BN, et al. c-Kit – The Novel Receptor: Physiological Roles, Downstream Signaling and Implications in Cancer. Jour Clin Med Res. 2021;2(3) :1-36.
Copyright© 2021 by Vajravelu BN, et al. All rights reserved. This is an open access article distributed under the terms of the Creative Commons Attribution License, which permits unrestricted use, distribution, and reproduction in any medium, provided the original author and source are credited.
Received 19 Sep, 2021 | Accepted 18 Oct, 2021 | Published 25 Oct, 2021 |
Abstract
The belief that adult mammalian heart lacks regenerative potential was challenged by the identification of c-kit positive Cardiac Progenitor Cells (CPCs) in the heart. SCIPIO; a phase I clinical trial; has shown that autologous c-kit positive CPCs improve cardiac function and quality of life when transplanted into ischemic heart disease patients. c-Kit is a type III receptor tyrosine kinase and a common stem cell antigen. Stem Cell Factor (SCF) is the only known ligand for c-kit. Activation of this membrane bound receptor is known to play a crucial role in many physiological processes like hematopoiesis, immune regulation, pigmentation, gametogenesis, intestinal motility, vasculogenesis, lung and brain development. Likewise, aberrant c-kit stimulation results in several pathological conditions, including tumors of the gut, blood, lung, breast, germ cells, melanocytes and mast cells. Several pro-growth, pro-survival signaling pathways like the MAPK, PI-3K and the phospholipase C and D pathways are involved in mediating the downstream effects, following the activation of this receptor. The goal of this article is to provide a comprehensive review of the structure, function, signaling mechanisms of c-kit activation and its role in normal and pathological conditions.
Keywords
Stem Cell Antigen; c-Kit Positive Cells; Cardiac Progenitor Cells; Stem Cell Factor, c-Kit Antigen
Introduction
Cellular Kit (c-kit) (also known as stem cell factor receptor or CD117) is a transmembrane glycoprotein with a molecular weight of 145 kDa [1-3]. Categorized as a type III receptor tyrosine kinase, c-kit was discovered in 1986 as the cellular homolog of the viral oncogene v-kit. It was identified to be the transforming gene of the Hardy-Zuckerman 4 feline sarcoma virus and hence named “kit”, an abbreviation for “kitten” [2]. Soon after the discovery of c-kit, studying the phenotype of c-kit mutants revealed that it is allelic with the dominant white spotting Locus (W) of mice [4]. A similar phenotype was found in mice with mutation in the Steel locus (SL) leading to the discovery of the only known ligand for c-kit called the steel factor or the Stem Cell Factor (SCF), the product of the Sl locus [5,6].
Structure of c-Kit
The c-kit protein has approximately 976 aminoacids and is comprised of five extracellular immunoglobulin-like (Ig-like) domains, a single transmembrane helix and a short cytoplasmic transmembrane domain (Fig. 1). The intracellular region of c-kit has two kinase domains separated by a short kinase insert and ends in the COOH terminus [2]. The c-kit gene is located in the chromosome 4q11 in humans and has 21 exons covering around 34 kb of DNA. Exon 1 encodes the signal peptide and translational initiation codon, exons 2-9 encode the five extra cellular Ig-like domains, exon 10 encodes the short transmembrane region and exons 11-21 encode the intracellular region comprising the two kinase domains separated by a short kinase insert and the terminal carboxy terminus [2,7]. c-Kit mRNA undergoes alternate splicing generating four isoforms in humans and two isoforms in mice. Two of the isoforms in humans differ by a short amino acid sequence GNNK. Although GNNK- is the predominant form, the GNNK+ and GNNK- are co-expressed in most tissues. Additionally, based on the presence of serine in the kinase insert, two more isoforms were identified [8-10]. Truncated kit (tr-kit), a shorter transcript, has only the second kinase domain and the carboxy terminus. This shorter transcript was found to play a critical role in gametogenesis by activating kinases of the Src Family and Phospholipase C (PLC γ), probably by acting as a scaffold [11,12]. Also, although the isoforms do not differ in their ligand binding capacity, it was found that they differ in their pattern of tyrosine phosphorylation, with the GNNK- isoform getting highly phosphorylated than its counterpart [13].
Figure 1: Schematic representation of the structure of c-kit: The type III receptor tyrosine kinase has five extracellular Ig-like domains, followed by a short transmembrane domain. The intracellular region has two kinase domain separated by a short kinase insert and ends in a COOH terminus. The Stem Cell Factor (SCF) is the only ligand that can bind to and activate c-kit.
Steel Factor or the Stem Cell Factor
Stem Cell Factor (SCF) or the steel factor is the only known ligand for c-kit. It exists as both a membrane bound isoform with 220 amino acids and as a soluble isoform with 248 amino acids (Fig. 2) [14]. The encoding gene is present on chromosome 12 in humans and has 9 exons producing two isoforms [15,16]. The isoforms differ in the presence or absence of exon 6 that has the cleavage site and both are initially produced as membrane bound proteins. The soluble isoform with exon 6 containing the cleavage site gets cleaved by proteases like Matrix Metalloproteases (MMPs), chymase-1 as well as ADAM family of proteases to yield a 165 amino acid soluble SCF which gets released in the extracellular space [17-19]. Both the isoforms are biologically active, however, they exhibit functional differences. Notably, the binding of soluble SCF to the c-kit receptor induces a rapid but transient activation of the receptor, whereas the membrane bound isoform has a more prolonged effect [20].
Several cell types express SCF and c-kit including endothelial cells, fibroblasts and bone marrow stromal cells where they serve roles in proliferation, survival, migration and differentiation [6,14,21]. However, the regulation of SCF expression is poorly understood.
Figure 2: Schematic representation of the structure of SCF: The ligand for c-kit, SCF has two isoforms; the membrane bound and the soluble isoform. Both are synthesized as a membrane bound form but the longer transcript gets cleaved by proteases due to the presence of the cleavage site, producing soluble SCF.
c-Kit Receptor Activation and Downregulation
Similar to other growth receptors, c-kit dimerizes upon ligand binding resulting in autophosphorylation and receptor activation [22]. The extra cellular Ig-like domain is critical for ligand binding and dimerization. SCF binds to c-kit at the first three Ig-like domains and induces a conformational change in the Ig-like domain 4 and 5 thereby bringing contiguous two c-kit monomers in close proximity. In its inactive state, the juxtamembrane domain of c-kit interacts with the kinase domain, thus inhibiting its enzyme activity [22-24]. However, upon dimerization, Tyr-568 and Tyr-570 in the juxtamembrane domain get phosphorylated, causing a conformational change which abolishes its inhibitory effect on the kinase domain. Subsequently, the kinase domain phosphorylates other tyrosine residues (autophosphorylation) on the opposite c-kit monomer, resulting in receptor activation and downstream signaling [24,25].
Upon continuous activation of c-kit, down regulation of c-kit occurs through three main processes
- Receptor internalization and degradation
- Negative regulation by protein kinase C
- Tyrosine dephosphorylation by phosphatases
- Receptor internalization and degradation: Following the binding of ligand and c-kit receptor activation, the E3 ubiquitin ligase c-Cbl, binds directly or indirectly through other proteins like Grb2, APS, p85 subunit of phospho inositol 3 kinase (PI3-kinase), CrkL [26-30]. Binding of this ubiquitin ligase leads to the covalent attachment of a single ubiquitin moiety on the receptor which marks it for internalization through clathrin coated pits followed by proteosomal and lysosomal degradation [31]. Internalization and degradation of the receptor after ligand binding is followed by the repopulation of new c-kit molecules at the membrane [32]. For the ubiquitination process to occur, the c-Cbl has to be phosphorylated and activated by the Src family kinases [26,33-35]. Recent studies have shown that other E3 ubiquitin ligases like SOCS6 are also involved in the downregulation process [36,37].
- Negative regulation by protein kinase C: The serine/threonine kinase protein kinase C (PKC) has been shown to play a role in c-kit inactivation [38-40]. SCF dependent c-kit activation results in a PI3-kinase dependent activation of phospholipase D leading to the release of phosphatidic acid. This in turn can be dephosphorylated forming Diacylglycerol (DAG), a potent activator of PKC. Activation of PKC leads to the phosphorylation of two critical serine residues present in the kinase insert of c-kit (S741 and S746). This causes inhibition of the receptor’s kinase activity and hence, termination of signal transduction [41].
- Tyrosine dephosphorylation by phosphatases: Receptor tyrosine kinases can also be inactivated by dephosphorylation of their tyrosine residues by phosphatases. It has been shown that the phosphatase SHP1 can interact with c-kit through one of its SH2 domain and dephosphorylates via its carboxy terminal protein tyrosine phosphatase domain [42, 43].
Signal Transduction
c-Kit activation is followed by the recruitment and activation of several downstream signaling molecules including Erk 1/2, Grb2, p38 MAPK, SFK, p85 subunit of PI3K and PLCγ) [44-46]. Fig. 3 depicts an overview of each of the main pathways involved in c-kit activation. It is important to remember that SCF dependent activation of c-kit can simultaneously activate more than one of these pathways. The combination of activated pathways and its downstream effects may differ depending on the species and on cell type (Fig. 3).
Figure 3: Signal Transduction in SCF Activated c-Kit Receptor: The ligand for c-kit, SCF binds to the c-kit receptor causing receptor dimerization and subsequent phosphorylation on key tyrosine residues. These residues act as a binding site for signal transduction molecules.
MAP Kinase Pathway
The Mitogen Activated Protein Kinase (MAPK) pathway is one of the major pathways activated by c-kit stimulation in melanocytes, mast cells and human leukemia cells [47-49]. The MAPK pathway is an essential pathway that promotes proliferation and survival of mammalian cells [50,51]. Although c-kit activation can activate all four types of MAP kinases namely ERK 1/2, p38 MAPK, ERK5 and JNK, activation of only ERK1/2 MAP kinase has been well characterized [52,53]. Ligand activated c-kit binds to the adapter protein Grb-2, which binds to the Guanine Nucleotide Exchange Factor (GEF) SOS. SOS then interacts with the membrane bound protein Ras and catalyzes the exchange of Ras-bound GDP with GTP. Subsequently, GTP activated Ras binds and activates several effector proteins including B-Raf. B-Raf activates Mek 1/2 kinase which in turn activates the pro-survival/pro-proliferation protein ERK, as evidenced by studies in melanoma cells [20,54]. Eventually, phosphorylated ERK 1/2 activates several downstream regulatory proteins including the ribosomal protein S6 Glycogen Synthase Kinase 3 (GSK3) and Microphthalmia Transcription Factor (MITF), thereby regulating cell survival and development [55-57].
PI3-Kinase Pathway
The PI3-kinase pathway is another important pathway activated by c-kit. Studies on SCF mediated c-kit activation in mast cells have shown that PI3K pathway is an important pathway regulating genes that are critical for cell survival, proliferation and differentiation [20,58]. Auto-phosphorylation of c-kit activates PI3K through phosphorylation of tyrosine 719 (Y721 in human) in mice [59]. Although there are four major classes of PI3 kinases, with the class I further divided into class 1A and 1B, much of the published articles address mainly class 1A. Structurally, class 1A PI3K has a regulatory subunit p85 and an enzymatic subunit p110, which can phosphorylate phosphoinositides [60]. p85 has SH2 domains through which it binds the phosphorylated c-kit receptor either directly at Tyr-712 or indirectly through the adapter protein GAB2 [61-64]. The binding induces a conformational change thereby activating the enzymatic p110 subunit. PI3K then translocates to the cell membrane and activates membrane bound Phosphoinositol 4, 5 bisphosphate (PIP2) to form Phosphoinositol 3, 4, 5 trisphosphate (PIP3). Then PIP3 activates proteins with Plekstrin Homology (PH) domains, including AKT. AKT is a serine/threonine kinase that regulates many downstream signaling proteins pertaining to growth, proliferation and survival [65,66]. It promotes cell survival by inactivating the pro-apoptotic protein Bad via phosphorylation [67]. AKT also promotes survival through promoting the proteosomal degradation of the fork head transcription factor (FoxO) proteins which would otherwise promote the expression of pro-apoptotic genes [68]. Furthermore, AKT activation has been shown to activate mammalian Target of Rapamycin (mTOR), which in turn activates ribosomal S6 kinase leading to the induction of pro-survival and anti-apoptotic genes [69,70].
SRC Family Kinase Pathway
The Src-Family Kinases (SFK) are a group of kinases that have been shown to mediate the effects of c-kit activation [52,71-73]. Some SFKs are ubiquitously expressed (Src, Yes, Fyn) whereas others are mostly expressed in hematopoietic cells (Lek, Hck, Fgr, Lyn, Blk). SFKs have an amino terminus, SH3 and SH2 domains that are involved in membrane targeting, a tyrosine kinase domain and a COOH-terminus. Inactive SH2 and the SH3 domains interact with the kinase domain and the phosphorylated COOH terminus, thus maintaining them in the inactive configuration. However, binding of the SH2 domain to a phosphorylated tyrosine residue or the SH3 domain to a proline rich region activates the enzyme and removes the inhibition of the kinase domain. The kinase activity of SFKs modulates the activity of multiple intracellular enzymes that play a role in cell proliferation, survival, differentiation and migration [74]. In addition, activation of SFKs regulates other survival/proliferation pathways including Erk 1/2, p38 MAPK, JNK and PI3K- AKT [52,71,72,73,75]. Indeed, SFKs have been shown to promote the proliferation of Mo7e cells, mast cells, melanocytes and also hematopoietic cells [73,76-78]. Interestingly, SFKs are also implicated in promoting the migration of primary hematopoietic cells like mast cells via phosphorylation of FAK and paxillin (a focal adhesion protein) [79-82].
Phospholipase C and D Pathways
There are 13 isoforms of Phospholipase C (PLC) [83-86] and two isoforms of phospholipase D (PLD) in humans [87,88]. Both these enzymes function to produce Diacylglycerol (DAG), a potent activator of the intracellular calcium releasing Protein Kinase C (PKC) that plays a significant role in cell proliferation, migration, survival [89]. Among the PLCs, the PLCγ has been extensively studied as a c-kit effector signaling pathway. PLCγ, which binds to Y730 residue on the activated c-kit receptor [46,90] hydrolyzes PIP2 to form Inositol 1, 4, 5 trisphosphate (IP3) and the membrane bound second messenger, DAG [91,92]. On the other hand, PLD produces DAG in an alternate route. It hydrolyzes the membrane lipid phosphatidylcholine or phosphatidylethanolamine to produce phosphatidic acid and choline. Phosphatidic acid hydrolase then hydrolyzes the phosphatidic acid to DAG. IP3 functions by mobilizing Ca2+ from the endoplasmic reticulum whereas DAG activates PKC [93]. The resulting increase in intracellular Ca2+ has been found to mediate many cellular events like migration, proliferation and apoptosis [94].
JAK/STAT Pathway
JAK/STAT pathway is involved in cellular proliferation as evidenced in fetal liver cells [95], as well as in other cellular functions like differentiation, survival and proliferation as shown in mast cells [96-98]. JAKs are cytoplasmic tyrosine kinases that are bound to the Receptor Tyrosine Kinases (RTKs). Upon ligand mediated receptor activation, JAKs phosphorylate more tyrosine residues on the receptor creating a binding site for proteins with SH2 domains, like STATs. Upon binding, STATs then are phosphorylated at their tyrosine residues by JAKs and subsequently form homo or hetero dimers. Dimerization of STATs are followed by their translocation into the nucleus, where they bind to the DNA through their DNA binding domains, thereby activating the transcription of genes that are involved in cellular proliferation, survival and differentiation [99].
Physiological Role of c-Kit
c-Kit plays an important role in regulating cellular characteristics of various cell types. For instance, Matsui et al. analyzed c-kit Signaling in vascular endothelial cells and found a significant role of c-kit in promoting survival, migration and differentiation [100]. Several studies have shown that c-kit participates in regulating the survival, maintenance, self-renewal and engraftment of hematopoietic stem cells [101-103]. In addition, a recent study has demonstrated that c-kit is involved in pancreatic islet development by promoting differentiation and proliferation of insulin and glucagon-producing cells [104-111]. The results from the study indicates a role of c-kit in normal hematopoiesis, pigmentation, gametogenesis, immune response, intestinal motility, vasculogenesis, lung compliance and brain development. Interestingly, c-kit mutations are also associated with various types of tumors such as mast cell tumors, Gastrointestinal Stromal Tumors (GIST) and leukemia, making it a proto-oncogene [112-114].
Hematopoiesis
SCF-c-kit signaling has an essential role in maintaining normal hematopoiesis. Homozygous c-kit mutations in mice are embryonically lethal due to the development of severe anemia [105-107]. Indeed, hematopoietic cells in the bone marrow express c-kit as early as day 8 post-gestation in the embryonic yolk sac. The expression increases until day 15 post-gestation and then gradually decreases [108]. In support of c-kit role in hematopoietic cells, a study showed that transplanting the bone marrow of c-kit-mutant mice could not replenish cellularity in irradiated hosts [109]. The bone marrow of these mutants were found to contain significantly less Colony Forming Units Spleen (CFU-S), Colony Forming Unit Erythroid (CFU-E) and Colony Forming Unit-Granulocyte-Macrophage (CFU-GM) when compared to their control littermates [110-113]. In a recent study by Kimura, et al., knocking out c-kit in adult mice using the Cre-lox system resulted in marked hematopoietic failure [114]. Furthermore, exogenous addition of SCF was able to promote the proliferation and survival of Hematopoietic Stem Cells (HSCs) with no effect on self- renewal [115]. Another study by Leary, et al., showed that SCF (in combination with IL-3) shortened the G0 phase in HSCs’ cell cycle and thus promoted their division [116]. A third study revealed that treating an enriched population of long-term repopulating hematopoietic stem cells increase their survival for at least 7 days compared to control cells [115]. Interestingly, SCF exhibits a synergistic effect with other cytokines like Erythropoietin (Epo), G-CSF, GM-CSF in promoting the proliferation and the colony size of BFU-E, CFU-GM and CFU-GEMM [117-119]. The findings of the aforementioned studies confirm that c-kit is essential for the maintenance and regeneration of Hematopoietic Stem and Progenitor Cells (HSPC). Not only does c-kit play a role in proliferation, but it is also involved in the interaction of HSPCs with the niche cells like the endothelial cells and the bone marrow stromal cells [114].
It is noteworthy that c-kit in the hematopoietic compartment is essential not only under steady state conditions, but also under conditions of stress, such as ischemia. For instance, Fazel, et al., have reported an increase in the myocardial expression of the c-kit ligand (SCF) following ischemic cardiac injury which led to the recruitment of hematopoietic progenitor cells to the site of injury and improved cardiac recovery. On the other hand, deletion of c-kit caused cardiac failure following the ischemic insult [120].
Hematopoiesis
SCF-c-kit signaling has an essential role in maintaining normal hematopoiesis. Homozygous c-kit mutations in mice are embryonically lethal due to the development of severe anemia [105-107]. Indeed, hematopoietic cells in the bone marrow express c-kit as early as day 8 post-gestation in the embryonic yolk sac. The expression increases until day 15 post-gestation and then gradually decreases [108]. In support of c-kit role in hematopoietic cells, a study showed that transplanting the bone marrow of c-kit-mutant mice could not replenish cellularity in irradiated hosts [109]. The bone marrow of these mutants were found to contain significantly less Colony Forming Units Spleen (CFU-S), Colony Forming Unit Erythroid (CFU-E) and Colony Forming Unit-Granulocyte-Macrophage (CFU-GM) when compared to their control littermates [110-113]. In a recent study by Kimura, et al., knocking out c-kit in adult mice using the Cre-lox system resulted in marked hematopoietic failure [114]. Furthermore, exogenous addition of SCF was able to promote the proliferation and survival of Hematopoietic Stem Cells (HSCs) with no effect on self- renewal [115]. Another study by Leary, et al., showed that SCF (in combination with IL-3) shortened the G0 phase in HSCs’ cell cycle and thus promoted their division [116]. A third study revealed that treating an enriched population of long-term repopulating hematopoietic stem cells increase their survival for at least 7 days compared to control cells [115]. Interestingly, SCF exhibits a synergistic effect with other cytokines like Erythropoietin (Epo), G-CSF, GM-CSF in promoting the proliferation and the colony size of BFU-E, CFU-GM and CFU-GEMM [117-119]. The findings of the aforementioned studies confirm that c-kit is essential for the maintenance and regeneration of Hematopoietic Stem and Progenitor Cells (HSPC). Not only does c-kit play a role in proliferation, but it is also involved in the interaction of HSPCs with the niche cells like the endothelial cells and the bone marrow stromal cells [114].
It is noteworthy that c-kit in the hematopoietic compartment is essential not only under steady state conditions, but also under conditions of stress, such as ischemia. For instance, Fazel, et al., have reported an increase in the myocardial expression of the c-kit ligand (SCF) following ischemic cardiac injury which led to the recruitment of hematopoietic progenitor cells to the site of injury and improved cardiac recovery. On the other hand, deletion of c-kit caused cardiac failure following the ischemic insult [120].
Dendritic Cells
Unlike in mast cells, the role of c-kit in Dendritic Cells (DC) is still unclear. It is however known that the expression of c-kit is maintained in mature DCs and mast cells unlike other mature cell types which lose c-kit upon differentiation [128, 129]. Studies have reported that treating DCs with Cholera Toxin (CT) or House Dust Mite (HDM) has resulted in increased expression of IL-6, which promoted the differentiation of DCs linked to c-kit expression [130]. c-Kit activation in DCs was associated with the activation of jagged-2, an interacting partner of Notch-2. Confirming the significance of c-kit and DCs, c-kit-mutant mice significantly reduced expression of IL-6 and jagged-2 resulting in defective differentiation of DCs [131].
Lymphopoiesis: Although the development and functions of B-lymphocytes are not affected by c-kit mutation, the development, proliferation and functions of T-lymphocytes are dependent on c-kit [132,133]. Indeed, very early precursors of the T-lymphocytes which are CD4-CD8-CD3- (Triple Negatives; TN) highly express c-kit [134]. Neonatal mice with mutations in c-kit were not able to contribute to thymopoiesis [135]. In addition, when these cells had their c-kit receptor blocked with an antibody, they were not able to properly reconstitute the T- cell compartment upon transplantation of thymic explants [134]. Also, SCF-c-kit signaling was found to potentiate the Mixed Lymphocyte Reaction (MLR) which is a measure of lymphocyte response to allogenic determinants presented by the antigen presenting cells of the host organism [136]. In combination with IL-7, SCF also increases the proliferation of early thymocytes [137]. Studies in c-kit mutant W/Wv mice have documented a defective intestinal immune system in these animals reiterating the importance of c-kit in the maturation of the intestinal immune system [138].
Pigmentation
The role of c-kit and SCF in the development and migration of melanocytes is well established. Luo, et al., have shown that SCF-dependent activation of c-kit in cultured melanocytes induces the transcription of the tyrosinase gene which is involved in melanogenesis [139]. Studies on the expression patterns of c-kit among different species have suggested that although to varying degrees, melanocytes are dependent on kit activation for the migration of their precursors (neural crest cells) and for their proliferation and survival [140]. Indeed, the neural crest cells in the head express c-kit as early as embryonic day 9.5. These precursors then migrate towards the eyes, inner ears and into the second brachial arch [141,142]. When kit is mutated, the migration of melanocyte precursors is blocked and they undergo apoptosis. Another study on mouse Hair Follicle (HF) melanocytes indicated that the proliferation of melanocytes is dependent on c-kit expression [143-145]. Interestingly, immunohistochemical analysis of HF melanocytes for Ki-67 and c-kit revealed that only the c-kit expressing subset were actively dividing. Also, SCF overexpression in mice significantly increased the number of actively dividing melanocytes and the use of a c-kit blocking antibody significantly reduced the number of these proliferating cells [143].
Gametogenesis
c-Kit and its ligand SCF play a crucial role in regulating reproduction in organisms. Phenotypic analysis of the White spotted and steel mice has revealed sterility of these mice due to a defect in the early development of Primordial Germ Cells (PGCs) which are required for normal spermatogenesis and oogenesis [146]. c-Kit mRNA expression can be detected in PGCs as early as 7 days post-coitum [147]. The SCF-c-kit signaling is involved in the proliferation and migration of PGCs from the hindgut to the genital ridge [148-150]. In the testes, c-kit expression can be detected in the postnatal interstitial cells of Leydig, primary spermatocytes and spermatogonia. Also, the truncated c-kit isoform (tr c-Kit) is found to be expressed in round spermatids [151]. Although this truncated c-kit contains no kinase activity as it has only the second kinase domain and the COOH terminal, it could cause the metaphase-anaphase transition when injected in mouse eggs [11]. Results of the study published by Packer, et al., have shown that the SCF-Kit signaling regulates the survival of germ cells in-vivo. Administration of an anti-c-kit antibody was found to promote apoptosis of primary spermatocytes and spermatogonia as identified by TUNEL staining [152]. On the other hand, in the post-natal ovary, c-kit is highly expressed in all oocytes at different stages of development. Using in-situ hybridization, the primordial oocytes were found to have a strong c-kit expression followed by interstitial theca cells [151]. The expression of both isoforms of SCF by the sertoli cells is critical for the survival and proliferation of spermatogonia. It is known that the c-kit protein expressed on spermatogonia interacts with the SCF expressed on sertoli cells leading to c-kit phosphorylation and activation [153]. Point mutation analysis of the c-kit receptor has identified that this activation leads to an increased proliferation of the differentiating type A spermatogonia through the activation of PI3K-AKT pathway [154].
Intestinal Motility
It is well known that loss of function mutations of the c-kit receptor in humans can cause piebaldism, a pigmentation disorder. In the same individuals, c-kit mutation also causes constipation due to the defective development of Interstitial Cells of Cajal (ICCs); the pacemaker cells of the intestine; responsible for its motility [155,156]. Interestingly, although these cells develop from the mesoderm, they establish an anatomic and functional connection with the enteric neurons during the later stages of their development [157]. The ICCs relay the electric impulses between the nerve cells of the intestine and the smooth muscles [156,158] and thus are required for the generation and propagation of autonomic gut motility. Defective development of the ICCs affects the enteric nervous system causing various dysfunctions. In the small intestine, c-kit+ paneth cells are the supporting cells that form the niche for the Lgr5+ intestinal stem cells. These paneth cells support the proliferation, survival and differentiation of the intestinal stem cells into secretory goblets or enteroendocrine cells. Similarly, the colon was found to have c-kit+ paneth-like goblet cells that support the Lgr5+ stem cells. These are the cells found at the base of the intestinal crypt that undergo cell division and differentiation to replace the epithelial lining of the colon [159]. c-Kit is also a key molecule in regulating mucosal immune responses. Upon stimulation with IL-1β, a subset of c-kit+ Innate Lymphoid Cells (ILCs) that are present in the intestine produces IL-22, an important cytokine secreted by the small intestinal cells to protect themselves against bacterial infection. This IL-22 was found to have a protective role against Inflammatory Bowel Disease (IBD) besides promoting the proliferation and survival of intestinal epithelial cells [160].
Vasculogenesis
c-Kit has an important role in vasculogenesis, under both physiological and pathological conditions. It was found to be important for the mobilization of hematopoietic and endothelial stem cells that are required for angiogenesis. A study by Heissig, et al., in a mouse model has illustrated that mobilization of stem cells upon injection of some chemotactic agents like SDF-1, G-CSF occurs through an induction of MMP-9 that increases the levels of soluble SCF in the blood [17]. In addition, SCF has a protective effect on Vascular Smooth Muscle Cells (VSMC) from oxidative stress induced apoptosis and it is also found to be essential for VSMC proliferation. For instance, published results of Wang, et al., have shown that treating VSMCs with SCF can significantly lower the amount of cleaved caspase-3 in hydrogen peroxide (H2O2) treated VSMCs [161]. This pro-survival effect of SCF on VSMCs was found to be mediated through activation of the PI3K-AKT pathway leading to upregulation of the anti-apoptotic protein Bcl-2 that acts by inhibiting the release of cytochrome-c from the mitochondria to the cytosol [161]. Besides playing a direct role in mobilizing cells that are required for angiogenesis, the kit ligand also has an indirect role through the activation of mast cells. For example, it was found that SCF can induce mast cells to release VEGF, a potent angiogenic factor [162]. Matsui, et al., have clearly established that treatment of HUVEC cells with SCF promoted its survival, migration and capillary tube formation through the downstream activation of both PI3K-AKT and ERK 1/2 pathways [100]. Also under normoxic conditions, the interaction of SCF with its receptor caused induction of hypoxia-inducible factor 1α (HIF-1 α), which enhances angiogenesis [163].
Lung Compliance
One of the critical functions of c-Kit was found to be the maintenance of lung compliance. Investigations in c-kit-deficient mice showed that the deficiency causes prominent enlargement of alveoli, a significant reduction in alveolar volume density and alveolar surface density, which are parameters of proper lung function. There was a significant alteration in the pressure-volume changes due to c-kit mutation with the c-kit mutant mice having a larger lung residual volume and static lung compliance [164]. Some of the abnormalities in the alveolar histology can be ascribed to a defective alveolar epithelium in which c-kit has been implicated to play a role in regulating its growth [164]. Also as discussed earlier, c-kit is essential for proper development, survival and maturation of HSCs, which contributes to the regeneration of the lung parenchyma following injury induced by radiation or by administering lipopolysaccharides, elastase and/or radiation [165-167]. In a murine model of elastase-induced emphysema, investigators found that instillation of hepatocyte growth factor repaired the injury and was accompanied by an increase in the levels of bone marrow derived c-kit+ cells, highlighting the beneficial reparative role of c-kit [168]. In another study by Ding, et al., the authors found that SCF-c-kit interaction can promote migration of c-kit+ bone marrow cells to the bleomycin injured lung and that migratory potential is reduced by inhibiting c-kit using imatinib (c-kit inhibitor) [169]. The same study found a subset of lung fibroblasts expressing c-kit which were highly responsive to SCF. Also co-culturing BM-derived c-kit+ cells with myofibrobalsts promoted their differentiation through a paracrine effect [169]. The findings of the above studies indicate that c-kit is required for the maintenance of normal alveolar architecture and that its expression on alveolar epithelial cells and lung fibroblasts is indispensable for proper maintenance of lung homeostasis.
Brain Development
Both c-kit and SCF are expressed widely in the nervous system. In most part of the nervous system, the c-kit receptor and its ligand are found to be expressed in neurons that are connected synaptically. In the embryo, the cells forming the floor of the neural tube were found to have prominent SCF expression, whereas the cells on the dorsal surface of the neural tube were strongly c-kit+ [170,171]. In the adult mouse brain, SCF expression is mostly confined to the Purkinje fibers in the cerebellum and the c-kit expression is found in the cells that are involved in synaptic connection with the Purkinje fibers namely, Golgi, stellate and basket cells. Apart from the cerebellum, a high level of SCF expression is also found in the thalamus and cerebral cortex, whereas high c-kit expression is found in the hippocampal region of the cerebral cortex [172]. In an in-vivo ischemic mouse brain model, SCF administration induced neurogenesis by promoting the proliferation of the neurons which was abolished by treating the cells with anti-c-kit antibody [173]. Neural stem/progenitor cells are important for the regeneration of the neural tissue after injury. In a ‘freeze’ brain injury model, SCF could cause migration of these neural stem/progenitor cells to the injured area both in vitro and in-vivo [174]. In the Dorsal Root Ganglia (DRG), c-kit expression can be detected on embryonic day 12.5 and persists during the post-natal period. c-Kit functions to support the survival of DRGs and SCF treatment can induce the growth of c-kit+ neuritis from the DAGs [175]. There are also reports suggesting that the c-kit receptor has a role in central and peripheral pain regulation [176].
Role in Tumorigenesis
The oncogenic role of c-kit is well established in a variety of tumors. Activating mutations of c-kit play a role in Gastrointestinal Stromal Tumors (GIST), germ cell tumors, malignant transformation of mast cells, breast tumors, malignant melanomas, small cell lung cancer and acute myeloid leukemia.
Gastrointestinal Stromal Tumors (GIST)
The interstitial cells of Cajal are the pacemaker of the intestine and have been implicated to be important for the motility of the intestine [156]. GISTs are tumors of the intestine that arise from these Cajal cells with most of them occurring in the stomach and the rest in the small intestine and rarely in the esophagus, colon, appendix and rectum [177-182]. Gain-of-function mutations of c-kit have been shown in approximately 95% of GISTs with the majority of these mutations being a deletion or a point mutation and occasionally an insertion [183]. These mutations result in constitutive activation of c-kit in the absence of its ligand, SCF. The intra-cellular juxta-membrane domain is the region where most of the mutations are reported to occur and is encoded by exon 11. The remaining mutations are reported to occur in the extra cellular region (exon 9) and in the intracellular kinase domain (exon 13) [184]. Mutations in the c-kit gene are classified as either enzymatic or regulatory in nature. The enzymatic mutations occur in the “enzymatic pocket” of the receptor affecting its enzymatic function whereas mutations in the intracellular juxta-membrane region which regulates the enzymatic pocket are considered regulatory [185]. GISTs with regulatory mutations of the c-kit receptor responds well to imatinib treatment than tumors with enzymatic c-kit mutations and hence have a better clinical prognosis [185]. The clinical symptoms of GISTs vary widely depending on the size of the tumor and the site of occurrence. The patients can be asymptomatic if the tumor is smaller than 2 cm or may present with abdominal discomfort, altered bowel habits or dysphagia. Larger tumors can cause abdominal obstruction, perforation, acute or chronic intestinal hemorrhage [179,180,186]. Untreated tumors can also metastasize to the live or abdomen and sometimes to the bones or lungs [186,187].
Germ Cell Tumors
SCF-c-kit Signaling is critical for normal spermatogenesis and oogenesis [148-150]. Mutations of c-kit have been found in Germ Cell Tumors (GCT) and are considered important in determining the pathogenesis and the response of the disease to treatment with imatinib [188-193]. GCTs are tumors of the primordial germ cells that form the male and female gametes. GCTs are broadly divided in to seminomas/dysgerminomas and nonseminomas/nondysgerminomas. Activating mutations of c-kit were identified in seminomas and dysgerminomas and were found to be not related to nonseminomas/nondysgerminomas [194,195]. Seminomas can be further classified in to unilateral and bilateral seminomas based on its occurrence. c-Kit mutations associated with these tumors were found to be Y823D, D816V, D816H and N822K. Almost all these mutations were detected in the intracellular kinase domain encoded by the exon 17 of the c-kit gene [196]. These mutations cause constitutive phosphorylation on the tyrosine residues of the receptor, thereby leading to prolonged activation of the downstream pro-survival and mitogenic signaling pathways, resulting in cellular transformation [192]. However, there are contradicting reports on the association of c-kit mutation in unilateral versus bilateral seminomas. Some studies have reported that mutations in c-kit are associated with bilateral seminomas [190,197] whereas others have reported no association [198]. The contradiction can be ascribed to differences in sample size or the mutation analysis technique used in each study. Like the seminomas, the dysgerminomas also have a strong association with c-kit expression. c-Kit overexpression was found in around 85% of dysgerminomas studied and 30% of those samples had a mutation in their c-kit gene [199]. More interestingly, c-kit overexpression was found to have a high correlation with advanced pathological stage of the disease [200].
Mast Cell Tumors
Mast cells are derived from CD34+ hematopoietic progenitor cells [201,202]. Immature mast cells reside in the bone marrow and peripheral blood [203]. They migrate to the tissues and differentiate to form mature mast cells. As mentioned in the discussion on the physiological role of c-kit, mast cell differentiation, survival, proliferation and migration is dependent on SCF-c-kit signaling [123-127]. Mast cells have intra cytoplasmic granules filled with pro-inflammatory and vasoactive mediators that are released upon IgE mediated immune response to an external stimulus [204]. The clinical features presented in mast cell neoplastic transformation (mastocytosis; MC) are essentially due to the release of these mediators in the blood or due to the scavenging of mast cells in various tissues. The World Health Organization (WHO) has classified MC under three major groups
- Cutaneous MC which is mostly prevalent among children and regresses as they reach adulthood
- Systemic MC (SM), which is further classified into indolent SM, SM with an associated clonal hematologic non-mast cell-like disease, aggressive SM and mast cell leukemia
- Extra cutaneous mastocytoma [205]. The D816V c-kit mutant was found to be associated with around 90% of the SM cases with the other mutations of exon 17 contributing to the remaining 10% [206-208].
It is important to remember that this mutation is not exclusively found in SM and hence cannot be used as a diagnostic criterion for SM. Studies in canine CM models have illuminated that c-kit mutation in CM is associated with increased proliferation and poor prognosis. Although activating mutations of c-kit is highly correlated with CM and SM, the expression of c-kit has no role in determining the pathogenesis of MC [209,210].
Breast Tumors
The role of c-kit in the pathogenesis of breast tumors is not well established and hence it is mostly of diagnostic and prognostic value. There are conflicting results on the expression of c-kit in tumors of the breast [211-215]. Most of the studies found an inverse relationship between c-kit expression and tumor transformation of breast tissue. There is a strong expression of c-kit in the normal mammary epithelium and in benign breast tumors. However, this expression gradually diminishes and is lost completely upon malignant transformation in females [211,212,216] . It is interesting to note that there is a difference in the expression of c-kit between male and female malignant breast conditions. Although the membrane and cytoplasmic expression of the receptor is absent in malignant breast tumors in females, a strong expression of c-kit has been identified in male malignant breast tissue samples. This indicates that c-kit has a pivotal role in the differentiation of mammary epithelium and is lost when the cells become more undifferentiated upon malignant transformation [216]. The difference in c-kit expression between male and female breast conditions indicates a significant difference in the molecular mechanisms of breast cancer development between genders. However, the pathways involved in the malignant transformation of human breast tissue are not clear. Unlike other tumors in which c-kit plays a role, none of the benign or malignant tumors of the breast are associated with mutations of c-kit [214,217].
Malignant Melanomas
SCF-c-kit axis also plays a critical role in the development, migration and proliferation of melanocytes [139-142,218]. Constitutively active c-kit promotes migration of melanocytes but also cause pigmentation and proliferation defects [219]. The reduction in pigmentation is due to increased degradation of the Micropthalmia- Associated Transcription Factor (MITF) caused by increased c-kit Signaling [54]. MITF is a transcription factor that is important for regulating the survival and proliferation of melanocytes [57]. Increased polyubiquitination and proteosomal degradation of MITF under constitutive c-kit activation reduces the survival of melanocytes and hence affects proper pigmentation [54]. Studies conducted early in the 1990s largely dismissed the role of SCF-c-kit axis in melanoma initiation and progression. These investigations highlighted the loss of c-kit expression when the melanoma become invasive and that expressing c-kit in invasive melanoma cell lines induces apoptosis and reduction in tumor growth [220-223]. However, later studies have shown a strong evidence of the involvement of c-kit in the transformation of melanocytes and in melanoma progression. These studies have highlighted that melanomas are highly heterogenous group of tumors. Although a majority of melanomas carry mutations in BRAF or NRAS leading to constitutive activation of the MAPK pathway and tumor transformation [224,225], there is a significant subset of melanomas that do not harbor BRAF or NRAS mutation but however, has a mutation in the c-kit gene [226,227]. A study conducted by Bastian showed that while BRAS and NRAS mutations are more common in melanomas occurring in areas with less sun exposure like the cutaneous melanomas, activating mutations of c-kit and gene amplification is more common in melanomas with chronic sun damage, acral and mucosal melanomas [226]. Subsequent studies have identified that 30% of c-kit mutations in melanoma are also accompanied by c-kit gene amplification and there are also reports of isolated gene amplification in melanomas without c-kit mutation, further confirming the heterogeneity in the pathway of tumor transformation in melanocytes [228-231].
Small Cell Lung Cancer
Small Cell Lung Cancer (SCLC) is an aggressive type of lung cancer contributing to 15-20% of all lung tumors. SSLCs have clinically poor prognosis and are not responsive to chemotherapy [232]. This necessitates finding better treatment strategies to benefit the patients suffering from the disease. For a better understanding of the disease and to find new treatment options, different molecular markers that were implicated in the pathogenesis of the disease were studied. One of such markers is the tyrosine kinase receptor, c-kit. The expression of c-kit on the SSLC cells analyzed using immunohistochemistry varies from 37% [233] to as high as 87% [234]. An interesting finding is that the tumor cells also co-express SCF [235,236] suggesting the existence of an autocrine loop playing a role in the tumor growth and survival. However, clinical studies conducted on patients with SCLC failed to support the above hypothesis. While some studies completely reject c-kit as a possible therapeutic target and a useful prognostic factor based on their results [237,238], other studies provide evidence to use c-kit as a marker to predict the clinical outcome [233,234,239]. The difference could be due to the sample size and the patient population selected for the study. Hence, the role of c-kit in SCLC is still debatable and warrants more clinical studies with the selection of appropriate patient populations.
Acute Myeloid Leukemia
Studies on the pathogenesis of Acute Myeloid Leukemia (AML) have identified two types of mutations that are responsible for the disease genesis. Type I includes the mutations of the receptor tyrosine kinases resulting in constitutive activation of the receptor and aberrant signal transduction leading to uncontrolled proliferation and survival of the transformed cells. Type II includes loss of function mutations of the transcription factors that are critical for the differentiation of myeloblasts [240]. Around 60-80% of myeloblasts express c-kit and mutations of c-kit are observed in around 17% of AML patients [241-244]. In core binding factor AML; a subtype of AML; around 52% of the patients have c-kit mutation [242], mostly in the extra cellular ligand binding domain encoded by exon 8 or in the kinase insert region encoded by exon 17. The only other RTK mutation that is observed at a higher frequency than c-kit is the mutation in FLT3 gene [245]. Although the role of c-kit in the pathogenesis of AML and as a prognostic factor is still unclear, several studies have suggested that mutations in the c-kit gene have an adverse outcome in AML patients [246-248]. Patients with c-kit mutations are reported to have higher relapse rate and poor prognosis, both in adults and in children [249-251].
c-KIT Inhibition in Cancer Treatment and Therapy Resistance
Among the currently available Tyrosine Kinase Inhibitors (TKIs), there are at least 16 drugs that are known to inhibit c-kit, albeit with variable potencies and specificities. This includes, axitinib, dovitinib, dasatinib, imatinib, motesanib diphosphate, pazopanib, sunitinib malate, masitinib, vatalanib, cabozantinib, tivozanib, amuvatinib, Ki8751, telatinib, dovitinib, tyrphoston [252]. These inhibitors competitively bind to c-kit and prevent the activation of downstream signaling cascade. Among the potent inhibitors of c-kit are dasatinib, sunitinib, masitinib and pazopanib[253, 254]. Most of these drugs also inhibit survival (BCR/ABL, FLT3), and/or angiogenesis (VEGF and PDGF) pathways. These drugs were initially designed to block pathways involved in malignant cell survival, proliferation and/or vasculogenesis, with the ultimate goal of combating cancer [255].
The question of using selective c-kit inhibitors to combat cancer has been debatable. However, while it is widely accepted that using selective c-kit inhibitors may result in blocking a pro-oncogenic pathway and should result in fewer side effects, there is increasing body of literature showing that proliferation and invasiveness of tumors are dependent on multiple pathways. This indicates that even if c-kit is inhibited by a small molecule, tumors may prove resistant to antineoplastic therapy via activating other proliferative pathways to keep growing. Thus, a multi-targeted therapy that inhibits multiple kinases are more likely to provide better clinical efficacy against tumors [256].
Another reason for cancer cell’s ability to resist the effect of c-kit inhibition by small molecules is the inability of these drugs to bind to the active conformational form of c-kit. For instance, although imatinib- a well-established tyrosine kinase inhibitor- has the ability to inhibit c-kit, some c-kit mutants were proved refractory to imatinib treatment because it was not able to bind active c-kit [255]. Thus, a new generation of c-kit inhibitors, such as sunitinib and regorafenib, have been designed to ensure their ability to bind both the non-activated and activated forms of c-kit [254,257,258]. Mutations in c-kit have also been implicated in inducing resistance of tumors to TKIs. For instance, GIST patients who are responsive to second generation sunitinib cease to respond to therapy if tumor cells acquire a D816H/V mutation in c-kit [259].
c-kit and Cancer Cell Stemness
Tumor recurrence either at the primary site or at the secondary site (following metastases) is still an unresolved issue in medical practice, leading, at times, to therapy failure. This resistance to therapy may be attributed to the activation of cancer stem cells in both sites. These cancer stem cells that remain after tumor resection are postulated to drive tumor recurrence in the primary cancer site. On the other hand, circulating cardiac stem cells are thought to drive recurrence in secondary sites. And because these cells are ultimately stem cells, they may also express c-kit on their cell membranes, just like regular non-oncogenic stem cells. Thus, it has been proposed that SCF/CD117 pro-survival and pro-proliferative signaling cascades on cancer stem cells may possibly play a role in promoting cancer progression despite chemotherapy, conferring resistance to treatment with antineoplastic agents [260,261].
In accordance, several reports have shown that c-kit is expressed in recurrent tumors [262-264] and that c-kit over-activation is seen in GIST, AML, mastocytosis and melanoma [265,266]. Also, it was shown that c-kit was expressed in 88% of surveyed cases where GIST had metastasized to bone [267]. In addition, higher c-kit expression in ovarian carcinomas appeared to result in poorer prognosis, peritoneal metastasis and shorter life expectancy [268]. Indeed, c-kit has been proposed to confer resistance to ovarian cancer cells against chemotherapy [269]. Also, c-kit expression in circulating cancer cells predicts advanced prostate cancer [262]. Increased expression of c-kit accompanied prostate cancer cells’ metastases to secondary sites; potentially through a downstream cross-talk that eventually resulted in BRCA2 downregulation in these cells [270]. In breast carcinomas and small cell lung cancers, c-kit was also a major regulator of cancerous cell survival, growth and proliferation [271].
Since c-kit activation in a cancer cell initiates multiple downstream signaling pathways that are believed to induce stemness (e.g. RAS/ERK, PI3-kinase, SRC, JAK/STAT and WNT), c-kit is thus implicated in maintaining cancer resilience. Indeed, it has been shown that activation of tyrosine kinase SRC in acute AML cells resulted in crosstalk with Akt-mTOR and promoted stemness of cells [272,273]. Similarly, Ras/ERK pathways have been implicated in conferring stemness to colon carcinoma and synovial sarcoma cell lines [274]. Taken together, these data suggests that c-kit is involved in cancer cell stemness and may be used as biomarker for cancer stem cells.
c-Kit Clinical Trials
The pleiotropic roles of c-kit as a protooncogene and a cell-surface marker has invited significant amount of research about its utility in cancer treatment and as a stem-cell marker through multiple clinical trials. Thus far, more than hundred clinical trials have been completed and more underway offering hope for better cancer treatments in the near future. Most of them have tested c-kit inhibitors like imatinib mesylate, nilotinib, dasatinib in cancers with constitutive c-kit activation such as melanomas, Gastrointestinal Stromal Tumors (GIST), acute myeloid leukemia, myelodysplastic syndromes and mastocytosis [275-278]. Few clinical trials have utilized c-kit positive stem cells for myocardial regeneration [279,280]. Although the outcomes of these clinical trials are promising, most of them still lack a more conclusive phase III testing.
Concluding Remarks
Since the discovery of c-kit in 1986, numerous studies have established its pivotal role in both physiological settings and in disease processes. RTK inhibitors such as imatinib has significantly improved the outcome of cancers due to c-kit mutation. However, there are still many areas that need further research. For example, any difference in the downstream effects of the splice variants of c-kit is largely unstudied and the problem of tumors developing resistance of c-kit inhibitors remain. A deeper understand about the interplay between specific c-kit mutation and the signal dysregulation is currently lacking. Also, whether c-kit binds or can be activated by a ligand other than SCF is unknown. Future studies focusing on elucidating these challenges will make this receptor a target for the treatment of various tumorous conditions.
Acknowledgement
We thank Dr. Kyung Hong for his guidance and Dr. Afsoon Moktar for the helpful discussion.
Conflict of Interest
The authors declare that they have no conflict of interest.
Ethical Approval
This article does not contain any studies with human participants or animals performed by any of the authors.
References
- Sherr CJ, Rettenmier CW, Sacca R, Roussel MF, Look AT, Stanley ER. The c-fms proto-oncogene product is related to the receptor for the mononuclear phagocyte growth factor, CSF 1. Cell. 1985;41(3):665-76.
- Yarden Y, Kuang WJ, Yang‐Feng T, Coussens L, Munemitsu S, Dull TJ, et al. Human proto‐oncogene c‐kit: a new cell surface receptor tyrosine kinase for an unidentified ligand. EMBO J. 1987;6(11):3341-51.
- Coussens L, Yang-Feng TL, Liao YC, Chen E, Gray A, McGrath J, et al. Tyrosine kinase receptor with extensive homology to EGF receptor shares chromosomal location with neu oncogene. Sci. 1985;230(4730):1132-9.
- Chabot B, Stephenson DA, Chapman VM, Besmer P, Bernstein A. The proto-oncogene c-kit encoding a transmembrane tyrosine kinase receptor maps to the mouse W locus. Nature. 1988;335(6185):88-9.
- Huang E, Nocka K, Beier DR, Chu TY, Buck J, Lahm HW, et al. The hematopoietic growth factor KL is encoded by the SI locus and is the ligand of the c-kit receptor, the gene product of the W locus. Cell. 1990;63(1):225-33.
- Zsebo KM, Williams DA, Geissler EN, Broudy VC, Martin FH, Atkins HL, et al. Stem cell factor is encoded at the SI locus of the mouse and is the ligand for the c-kit tyrosine kinase receptor. Cell. 1990;63(1):213-24.
- Copeland NG, Gilbert DJ, Cho BC, Donovan PJ, Jenkins NA, Cosman D, et al. Mast cell growth factor maps near the steel locus on mouse chromosome 10 and is deleted in a number of steel alleles. Cell. 1990;63(1):175-83.
- Crosier PS, Ricciardi ST, Hall LR, Vitas MR, Clark SC, Crosier KE. Expression of isoforms of the human receptor tyrosine kinase c-kit in leukemic cell lines and acute myeloid leukemia. Blood. 1993;82(4):1151-8.
- Reith AD, Ellis C, Lyman SD, Anderson DM, Williams DE, Bernstein A, et al. Signal transduction by normal isoforms and W mutant variants of the Kit receptor tyrosine kinase. The EMBO J. 1991;10(9):2451-9.
- Zhu WM, Dong WF, Minden M. Alternate splicing creates two forms of the human kit protein. Leukemia Lymphoma. 1994;12(5-6):441-7.
- Paronetto MP, Venables JP, Elliott DJ, Geremia R, Rossi P, Sette C. tr-kit promotes the formation of a multimolecular complex composed by Fyn, PLC γ 1 and Sam68. Oncogene. 2003;22(54):8707-15.
- Rossi P, Marziali G, Albanesi C, Charlesworth A, Geremia R, Sorrentino V. A novel c-kit transcript, potentially encoding a truncated receptor, originates within a kit gene intron in mouse spermatids. Dev Bio. 1992;152(1):203-7.
- Caruana G, Cambareri AC, Ashman LK. Isoforms of c-KIT differ in activation of signalling pathways and transformation of NIH3T3 fibroblasts. Oncogene. 1999;18(40):5573-81.
- Anderson DM, Williams DE, Tushinski R, Gimpel S, Eisenman J, Cannizzaro LA, et al. Alternate splicing of mRNAs encoding human mast cell growth factor and localization of the gene to chromosome 12q22-q24. Cell Growth Differ. 1991;2(8):373-8.
- Martin FH, Suggs SV, Langley KE, Lu HS, Ting J, Okino KH, et al. Primary structure and functional expression of rat and human stem cell factor DNAs. Cell. 1990;63(1):203-11.
- Ropers HH, Craig IW. Report of the committee on the genetic constitution of chromosomes 12 and 13. Cytogen Gen Res. 1989;51(1-4):259-79.
- Heissig B, Hattori K, Dias S, Friedrich M, Ferris B, Hackett NR, et al. Recruitment of stem and progenitor cells from the bone marrow niche requires MMP-9 mediated release of kit-ligand. Cell. 2002;109(5):625-37.
- Longley BJ, Tyrrell L, Ma Y, Williams DA, Halaban R, Langley K, et al. Chymase cleavage of stem cell factor yields a bioactive, soluble product. Proceed Nat Acad Sci. 1997;94(17):9017-21.
- Zou J, Zhu F, Liu J, Wang W, Zhang R, Garlisi CG, et al. Catalytic activity of human ADAM33. J Biol Chem. 2004;279(11):9818-30.
- Rönnstrand L. Signal transduction via the stem cell factor receptor/c-Kit. Cellular and Molecular Life Sciences CMLS. 2004;61(19):2535-48.
- Heinrich MC, Dooley DC, Freed AC, Band L, Hoatlin ME, Keeble WW, Peters ST, Silvey KV, Ey FS, Kabat D. Constitutive expression of steel factor gene by human stromal cells. Blood. 1993;82(3):771-83.
- Lemmon MA, Schlessinger J. Cell signaling by receptor tyrosine kinases. Cell. 2010;141(7):1117-34.
- Yuzawa S, Opatowsky Y, Zhang Z, Mandiyan V, Lax I, Schlessinger J. Structural basis for activation of the receptor tyrosine kinase KIT by stem cell factor. Cell. 2007;130(2):323-34.
- Mol CD, Dougan DR, Schneider TR, Skene RJ, Kraus ML, Scheibe DN, et al. Structural basis for the autoinhibition and STI-571 inhibition of c-Kit tyrosine kinase. J Biol Chem. 2004;279(30):31655-63.
- Mol CD, Lim KB, Sridhar V, Zou H, Chien EY, Sang BC, et al. Structure of a c-kit product complex reveals the basis for kinase transactivation. J Biol Chem. 2003;278(34):31461-4.
- Masson K, Heiss E, Band H, Rönnstrand L. Direct binding of Cbl to Tyr568 and Tyr936 of the stem cell factor receptor/c-Kit is required for ligand-induced ubiquitination, internalization and degradation. Biochem J. 2006;399(1):59-67.
- Sattler M, Salgia R, Shrikhande G, Verma S, Pisick E, Prasad KV, et al. Steel factor induces tyrosine phosphorylation of CRKL and binding of CRKL to a complex containing c-Kit, phosphatidylinositol 3-kinase, and p120CBL. J Biol Chem. 1997;272(15):10248-53.
- Sun J, Pedersen M, Bengtsson S, Rönnstrand L. Grb2 mediates negative regulation of stem cell factor receptor/c-Kit signaling by recruitment of Cbl. Exp Cell Res. 2007;313(18):3935-42.
- Wisniewski D, Strife A, Clarkson B. c-kit ligand stimulates tyrosine phosphorylation of the c-Cbl protein in human hematopoietic cells. Leukemia. 1996;10(9):1436-42.
- Wollberg P, Lennartsson J, Gottfridsson E, Yoshimura A, Rönnstrand L. The adapter protein APS associates with the multifunctional docking sites Tyr-568 and Tyr-936 in c-Kit. Biochemical J. 2003;370(3):1033-8.
- Haglund K, Sigismund S, Polo S, Szymkiewicz I, Di Fiore PP, Dikic I. Multiple monoubiquitination of RTKs is sufficient for their endocytosis and degradation. Nat Cell Bio. 2003;5(5):461-6.
- Shimizu Y, Ashman LK, Du Z, Schwartz LB. Internalization of Kit together with stem cell factor on human fetal liver-derived mast cells: new protein and RNA synthesis are required for reappearance of kit. J Immunol. 1996;156(9):3443-9.
- Broudy VC, Lin NL, Liles WC, Corey SJ, O’Laughlin B, Mou S, Linnekin D. Signaling via Src family kinases is required for normal internalization of the receptor c-Kit. Blood. J Am Soc Hematol. 1999;94(6):1979-86.
- Jahn T, Seipel P, Coutinho S, Urschel S, Schwarz K, Miething C, et al. Analysing c-kit internalization using a functional c-kit-EGFP chimera containing the fluorochrome within the extracellular domain. Oncogene. 2002;21(29):4508-20.
- Voytyuk O, Lennartsson J, Mogi A, Caruana G, Courtneidge S, Ashman LK, Ronnstrand L. Src family kinases are involved in the differential signaling from two splice forms of c-Kit. J Biol Chem. 2003;278(11):9159-66.
- Bayle J, Lopez S, Iwaï K, Dubreuil P, De Sepulveda P. The E3 ubiquitin ligase HOIL-1 induces the polyubiquitination and degradation of SOCS6 associated proteins. FEBS Lett. 2006;580(11):2609-14.
- Zadjali F, Pike AC, Vesterlund M, Sun J, Wu C, Li SS, et al. Structural basis for c-KIT inhibition by the suppressor of cytokine signaling 6 (SOCS6) ubiquitin ligase. J Biol Chem. 2011;286(1):480-90.
- Blume‐Jensen P, Siegbahn A, Stabel S, Heldin CH, Rönnstrand L. Increased Kit/SCF receptor induced mitogenicity but abolished cell motility after inhibition of protein kinase C. The EMBO J. 1993;12(11):4199-209.
- Blume-Jensen P, Wernstedt C, Heldin CH, Rönnstrand L. Identification of the Major Phosphorylation Sites for Protein Kinase C in Kit/Stem Cell Factor Receptor in Vitro and in Intact Cells. J Biol Chem. 1995;270(23):14192-200.
- Edling CE, Pedersen M, Carlsson L, Rönnstrand L, Palmer RH, Hallberg B. Haematopoietic progenitor cells utilise conventional PKC to suppress PKB/Akt activity in response to c‐Kit stimulation. Br J Haematol. 2007;136(2):260-8.
- Yee NS, Hsiau CW, Serve H, Vosseller K, Besmer P. Mechanism of down-regulation of c-kit receptor. Roles of receptor tyrosine kinase, phosphatidylinositol 3′-kinase, and protein kinase C. J Biol Chem. 1994;269(50):31991-8.
- Kozlowski M, Larose L, Lee F, Le DM, Rottapel R, Siminovitch KA. SHP-1 binds and negatively modulates the c-Kit receptor by interaction with tyrosine 569 in the c-Kit juxtamembrane domain. Mol Cell Bio. 1998;18(4):2089-99.
- Paulson RF, Vesely S, Siminovitch KA, Bernstein A. Signalling by the W/Kit receptor tyrosine kinase is negatively regulated in vivo by the protein tyrosine phosphatase Shp1. Nat Gen. 1996;13(3):309-15.
- Thömmes K, Lennartsson J, CARLBERG M, Rönnstrand L. Identification of Tyr-703 and Tyr-936 as the primary association sites for Grb2 and Grb7 in the c-Kit/stem cell factor receptor. Biochem J. 1999;341(1):211-6.
- Lennartsson J, Wernstedt C, Engström U, Hellman U, Rönnstrand L. Identification of Tyr900 in the kinase domain of c-Kit as a Src-dependent phosphorylation site mediating interaction with c-Crk. Exp Cell Res. 2003;288(1):110-8.
- Gommerman JL, Sittaro D, Klebasz NZ, Williams DA, Berger SA. Differential stimulation of c-Kit mutants by membrane-bound and soluble Steel Factor correlates with leukemic potential. Blood. J Am Soc Hematol. 2000;96(12):3734-42.
- McDaniel AS, Allen JD, Park SJ, Jaffer ZM, Michels EG, Burgin SJ, Chen S, Bessler WK, Hofmann C, Ingram DA, Chernoff J. Pak1 regulates multiple c-Kit mediated Ras-MAPK gain-in-function phenotypes in Nf1+/− mast cells. Blood. J Am Soc Hematol. 2008;112(12):4646-54.
- Marschall JS, Wilhelm T, Schuh W, Huber M. MEK/Erk-based negative feedback mechanism involved in control of Steel Factor-triggered production of Krüppel-like factor 2 in mast cells. Cell Signal. 2012;24(4):879-88.
- Buet D, Gallais I, Lauret E, Denis N, Lombard B, Guillonneau F, et al. Cotargeting signaling pathways driving survival and cell cycle circumvents resistance to Kit inhibitors in leukemia. Blood. J Am Soc Hematol. 2012;119(18):4228-41.
- Ballif BA, Blenis J. Molecular mechanisms mediating mammalian mitogen-activated protein kinase (MAPK) kinase (MEK)-MAPK cell survival signals. Cell Growth Different. 2001;12(8):397-408.
- Pearson G, Robinson F, Beers Gibson T, Xu BE, Karandikar M, Berman K, et al. Mitogen-activated protein (MAP) kinase pathways: regulation and physiological functions. Endocrine Rev. 2001;22(2):153-83.
- Lennartsson J, Blume-Jensen P, Hermanson M, Pontén E, Carlberg M, RoÈnnstrand L. Phosphorylation of Shc by Src family kinases is necessary for stem cell factor receptor/c-kit mediated activation of the Ras/MAP kinase pathway and c-fos induction. Oncogene. 1999;18(40):5546-53.
- Simon C, Dondi E, Chaix A, De Sepulveda P, Kubiseski TJ, Varin-Blank N, et al. Lnk adaptor protein down-regulates specific Kit-induced signaling pathways in primary mast cells. Blood. J Am Soc Hematol. 2008;112(10):4039-47.
- Hemesath TJ, Price ER, Takemoto C, Badalian T, Fisher DE. MAP kinase links the transcription factor Microphthalmia to c-Kit signalling in melanocytes. Nat. 1998;391(6664):298-301.
- Pende M, Um SH, Mieulet V, Sticker M, Goss VL, Mestan J, et al. S6K1−/−/S6K2−/− mice exhibit perinatal lethality and rapamycin-sensitive 5′-terminal oligopyrimidine mRNA translation and reveal a mitogen-activated protein kinase-dependent S6 kinase pathway. Mol Cell Bio. 2004;24(8):3112-24.
- Saito Y, Vandenheede JR, Cohen P. The mechanism by which epidermal growth factor inhibits glycogen synthase kinase 3 in A431 cells. Biochem J. 1994;303(1):27-31.
- Levy C, Khaled M, Fisher DE. MITF: master regulator of melanocyte development and melanoma oncogene. Trends Mol Med. 2006;12(9):406-14.
- Krishnan S, Mali RS, Koehler KR, Vemula S, Chatterjee A, Ghosh J, et al. Class IA PI3Kinase Regulatory Subunit, p85α, Mediates Mast Cell Development through Regulation of Growth and Survival Related Genes. PloS One. 2012;7(1):e28979.
- Ueda S, Mizuki M, Ikeda H, Tsujimura T, Matsumura I, Nakano K, et al. Critical roles of c-Kit tyrosine residues 567 and 719 in stem cell factor-induced chemotaxis: contribution of src family kinase and PI3-kinase on calcium mobilization and cell migration. Blood. J Am Soc Hematol. 2002;99(9):3342-9.
- Kirshenbaum AS, Goff JP, Semere T, Foster B, Scott LM, Metcalfe DD. Demonstration that human mast cells arise from a progenitor cell population that is CD34+, c-kit+, and expresses aminopeptidase N (CD13). Blood. J Am Soc Hematol. 1999;94(7):2333-42.
- Serve H, Hsu YC, Besmer P. Tyrosine residue 719 of the c-kit receptor is essential for binding of the P85 subunit of phosphatidylinositol (PI) 3-kinase and for c-kit-associated PI 3-kinase activity in COS-1 cells. J Biol Chem. 1994;269(8):6026-30.
- Lev S, Givol D, Yarden Y. Interkinase domain of kit contains the binding site for phosphatidylinositol 3’kinase. Proc Natl Acad Sci.1992;89(2):678-82.
- Nishida K, Wang L, Morii E, Park SJ, Narimatsu M, Itoh S, Yamasaki S, Fujishima M, Ishihara K, Hibi M, Kitamura Y. Requirement of Gab2 for mast cell development and KitL/c-Kit signaling. Blood. J Am Soc Hematol. 2002;99(5):1866-9.
- Yu M, Luo J, Yang W, Wang Y, Mizuki M, Kanakura Y, et al. The scaffolding adapter Gab2, via Shp-2, regulates kit-evoked mast cell proliferation by activating the Rac/JNK pathway. J Biol Chem. 2006;281(39):28615-26.
- Klippel A, Escobedo JA, Hirano M, Williams LT. The interaction of small domains between the subunits of phosphatidylinositol 3-kinase determines enzyme activity. Mol Cell Bio. 1994;14(4):2675-85.
- Manning BD, Cantley LC. AKT/PKB signaling: navigating downstream. Cell. 2007;129(7):1261-74.
- Blume-Jensen P, Janknecht R, Hunter T. The kit receptor promotes cell survival via activation of PI 3-kinase and subsequent Akt-mediated phosphorylation of Bad on Ser136. Curr Bio. 1998;8(13):779-85.
- Brunet A, Bonni A, Zigmond MJ, Lin MZ, Juo P, Hu LS, et al. Akt promotes cell survival by phosphorylating and inhibiting a Forkhead transcription factor. Cell. 1999;96(6):857-68.
- Feng LX, Ravindranath N, Dym M. Stem cell factor/c-kit up-regulates cyclin D3 and promotes cell cycle progression via the phosphoinositide 3-kinase/p70 S6 kinase pathway in spermatogonia. J Bio Chem. 2000;275(33):25572-6.
- Kim MS, Kuehn HS, Metcalfe DD, Gilfillan AM. Activation and function of the mTORC1 pathway in mast cells. J Immunol. 2008;180(7):4586-95.
- Arcaro A, Aubert M, Del Hierro ME, Khanzada UK, Angelidou S, Tetley TD, et al. Critical role for lipid raft-associated Src kinases in activation of PI3K-Akt signalling. Cellular Signalling. 2007;19(5):1081-92.
- Bondzi C, Litz J, Dent P, Krystal GW. Src family kinase activity is required for Kit-mediated mitogen-activated protein (MAP) kinase activation, however loss of functional retinoblastoma protein makes MAP kinase activation unnecessary for growth of small cell lung cancer cells. Cell Growth Differ. 2000;11(6):305-14.
- Timokhina I, Kissel H, Stella G, Besmer P. Kit signaling through PI 3‐kinase and Src kinase pathways: an essential role for Rac1 and JNK activation in mast cell proliferation. EMBO J. 1998;17(21):6250-62.
- Bromann PA, Korkaya H, Courtneidge SA. The interplay between Src family kinases and receptor tyrosine kinases. Oncogene. 2004;23(48):7957-68.
- Samayawardhena LA, Hu J, Stein PL, Craig AW. Fyn kinase acts upstream of Shp2 and p38 mitogen-activated protein kinase to promote chemotaxis of mast cells towards stem cell factor. Cell Signalling. 2006;18(9):1447-54.
- Linnekin D, DeBerry CS, Mou S. Lyn associates with the juxtamembrane region of c-Kit and is activated by stem cell factor in hematopoietic cell lines and normal progenitor cells. J Biol Chem. 1997;272(43):27450-5.
- Mou S, Linnekin D. Lyn is activated during late G1 of stem-cell-factor-induced cell cycle progression in haemopoietic cells. Biochem J. 1999;342(1):163-70.
- Phung B, Sun J, Schepsky A, Steingrimsson E, Rönnstrand L. C-KIT signaling depends on microphthalmia-associated transcription factor for effects on cell proliferation. PloS One. 2011;6(8):e24064.
- O’Laughlin-Bunner B, Radosevic N, Taylor ML, DeBerry C, Metcalfe DD, Zhou M, Lowell C, Linnekin D. Lyn is required for normal stem cell factor–induced proliferation and chemotaxis of primary hematopoietic cells. Blood. J Am Soc Hematol. 2001;98(2):343-50.
- Sieg DJ, Hauck CR, Ilic D, Klingbeil CK, Schaefer E, Damsky CH, et al. FAK integrates growth-factor and integrin signals to promote cell migration. Nat Cell Bio. 2000;2(5):249-56.
- Thomas SM, Soriano P, Imamoto A. Specific and redundant roles of Src and Fyn in organizing the cytoskeleton. Nat. 1995;376(6537):267-71.
- Glenney JR, Zokas L. Novel tyrosine kinase substrates from Rous sarcoma virus-transformed cells are present in the membrane skeleton. J Cell Bio. 1989;108(6):2401-8.
- Lopez I, Mak EC, Ding J, Hamm HE, Lomasney JW. A novel bifunctional phospholipase C that is regulated by Gα12 and stimulates the Ras/mitogen-activated protein kinase pathway. J Biol Chem. 2001;276(4):2758-65.
- Rhee SG, Bae YS. Regulation of phosphoinositide-specific phospholipase C isozymes. J Biol Chem. 1997;272(24):15045-8.
- Rhee SG, Suh PG, Ryu SH, Lee SY. Studies of inositol phospholipid-specific phospholipase C. Sci. 1989;244(4904):546-50.
- Song C, Hu CD, Masago M, Kariya KI, Yamawaki-Kataoka Y, Shibatohge M, et al. Regulation of a novel human phospholipase C, PLCε, through membrane targeting by Ras. J Biol Chem. 2001;276(4):2752-7.
- Colley WC, Sung TC, Roll R, Jenco J, Hammond SM, Altshuller Y, et al. Phospholipase D2, a distinct phospholipase D isoform with novel regulatory properties that provokes cytoskeletal reorganization. Curr Biol. 1997;7(3):191-201.
- Hammond SM, Altshuller YM, Sung TC, Rudge SA, Rose K, Engebrecht J, et al. Human ADP-ribosylation factor-activated phosphatidylcholine-specific phospholipase D defines a new and highly conserved gene family (∗). J Biol Chem. 1995;270(50):29640-3.
- Divecha N, Irvine RF. Phospholipid signaling. Cell. 1995;80(2):269-78.
- Trieselmann NZ, Soboloff J, Berger SA. Mast cells stimulated by membrane-bound, but not soluble, steel factor are dependent on phospholipase C activation. CMLS. 2003;60(4):759-66.
- Wright TM, Rangan LA, Shin HS, Raben DM. Kinetic analysis of 1, 2-diacylglycerol mass levels in cultured fibroblasts. Comparison of stimulation by alpha-thrombin and epidermal growth factor. J Biol Chem. 1988;263(19):9374-80.
- Cook SJ, Palmer S, Plevin R, Wakelam MJ. Mass measurement of inositol 1, 4, 5-trisphosphate and sn-1, 2-diacylglycerol in bombesin-stimulated Swiss 3T3 mouse fibroblasts. Biochemical J. 1990;265(2):617-20.
- Exton JH. Phosphatidylcholine breakdown and signal transduction. Biochim Biophysica Acta (BBA)-Lipids and Lipid Metabolism. 1994;1212(1):26-42.
- Berridge MJ, Lipp P, Bootman MD. The versatility and universality of calcium signalling. Nat Rev Mol Cell Bio. 2000;1(1):11-21.
- Parganas E, Wang D, Stravopodis D, Topham DJ, Marine JC, Teglund S, et al. Jak2 is essential for signaling through a variety of cytokine receptors. Cell. 1998;93(3):385-95.
- Okayama Y, Kawakami T. Development, migration, and survival of mast cells. Immunologic Res. 2006;34(2):97-115.
- Ryan JJ, Huang H, McReynolds LJ, Shelburne C, Hu-Li J, Huff TF, et al. Stem cell factor activates STAT-5 DNA binding in IL-3-derived bone marrow mast cells. Exp Hematol. 1997;25(4):357-62.
- Shelburne CP, McCoy ME, Piekorz R, Sexl VV, Gillespie SR, Bailey DP, et al. Stat5: an essential regulator of mast cell biology. Mol Immunol. 2002;38(16-18):1187-91.
- Heim MH. The Jak-STAT pathway: cytokine signalling from the receptor to the nucleus. J Recep Signal Transd. 1999;19(1-4):75-120.
- Matsui J, Wakabayashi T, Asada M, Yoshimatsu K, Okada M. Stem cell factor/c-kit signaling promotes the survival, migration, and capillary tube formation of human umbilical vein endothelial cells. J Biol Chem. 2004;279(18):18600-7.
- Thorén LA, Liuba K, Bryder D, Nygren JM, Jensen CT, Qian H, et al. Kit regulates maintenance of quiescent hematopoietic stem cells. J Immunol. 2008;180(4):2045-53.
- Kent D, Copley M, Benz C, Dykstra B, Bowie M, Eaves C. Regulation of hematopoietic stem cells by the steel factor/KIT signaling pathway. Clin Can Res. 2008;14(7):1926-30.
- Cosgun KN, Rahmig S, Mende N, Reinke S, Hauber I, Schäfer C, et al. Kit regulates HSC engraftment across the human-mouse species barrier. Cell Stem Cell. 2014;15(2):227-38.
- Wu Y, Li J, Saleem S, Yee SP, Hardikar AA, Wang R. c-Kit and stem cell factor regulate PANC-1 cell differentiation into insulin-and glucagon-producing cells. Lab Invest. 2010;90(9):1373-84.
- Geissler EN, McFarland EC, Russell ES. Analysis of pleiotropism at the dominant white-spotting (W) locus of the house mouse: a description of ten new W alleles. Genetics. 1981;97(2):337-61.
- Nocka K, Tan JC, Chiu E, Chu TY, Ray P, Traktman P, Besmer P. Molecular bases of dominant negative and loss of function mutations at the murine c‐kit/white spotting locus: W37, Wv, W41 and W. EMBO J. 1990;9(6):1805-13.
- Reith AD, Rottapel R, Giddens E, Brady C, Forrester L, Bernstein A. W mutant mice with mild or severe developmental defects contain distinct point mutations in the kinase domain of the c-kit receptor. Genes Dev. 1990;4(3):390-400.
- Palacios R, Nishikawa SL. Developmentally regulated cell surface expression and function of c-kit receptor during lymphocyte ontogeny in the embryo and adult mice. Dev. 1992;115(4):1133-47.
- Russell ES, Bernstein SE, Lawson FA, Smith LJ. Long-continued function of normal blood-forming tissue transplanted into genetically anemic hosts. J Nat Can Inst. 1959;23(3):557-66.
- McCulloch EA, Siminovitch L, Till JE. Spleen-colony formation in anemic mice of genotype WW. Sci. 1964;144(3620):844-6.
- Lewis JP, O’GRADY LF, Bernstein SE, Russell ES, Trobaugh Jr FE. Growth and differentiation of transplanted W/Wv marrow. Blood. 1967;30(5):601-16.
- Gregory CJ, Eaves AC. Three stages of erythropoietic progenitor cell differentiation distinguished by a number of physical and biologic properties. Blood. 1978;51(3):527-37.
- Barker JE. Sl/Sld hematopoietic progenitors are deficient in situ. Exp Hematol. 1994;22(2):174-7.
- Kimura Y, Ding B, Imai N, Nolan DJ, Butler JM, Rafii S. c-Kit-mediated functional positioning of stem cells to their niches is essential for maintenance and regeneration of adult hematopoiesis. PloS One. 2011;6(10):e26918.
- Li CL, Johnson GR. Stem cell factor enhances the survival but not the self-renewal of murine hematopoietic long-term repopulating cells. Blood. 1994;84(2):408-14.
- Leary AG, Zeng HQ, Clark SC, Ogawa M. Growth factor requirements for survival in G0 and entry into the cell cycle of primitive human hemopoietic progenitors. Proc Nat Acad Sci. 1992;89(9):4013-7.
- Bernstein ID, Andrews RG, Zsebo KM. Recombinant human stem cell factor enhances the formation of colonies by CD34+ and CD34+ lin-cells, and the generation of colony-forming cell progeny from CD34+ lin-cells cultured with interleukin-3, granulocyte colony-stimulating factor, or granulocyte-macrophage colony-stimulating factor. Blood. 1991;77(11):2316-21.
- McNiece IK, Langley KE, Zsebo KM. Recombinant human stem cell factor synergises with GM-CSF, G-CSF, IL-3 and epo to stimulate human progenitor cells of the myeloid and erythroid lineages. Experimental Hematol. 1991;19(3):226-31.
- Nocka K, Buck J, Levi E, Besmer P. Candidate ligand for the c‐kit transmembrane kinase receptor: KL, a fibroblast derived growth factor stimulates mast cells and erythroid progenitors. The EMBO J. 1990;9(10):3287-94.
- Fazel SS, Chen L, Angoulvant D, Li SH, Weisel RD, Keating A, et al. Activation of c‐kit is necessary for mobilization of reparative bone marrow progenitor cells in response to cardiac injury. The FASEB J. 2008;22(3):930-40.
- Kitamura Y, Go S, Hatanaka K. Decrease of mast cells in W/Wv mice and their increase by bone marrow transplantation. Blood. 1978;52(2):447-52.
- Lemura A, Tsai M, Ando A, Wershil BK, Galli SJ. The c-kit ligand, stem cell factor, promotes mast cell survival by suppressing apoptosis. Am J Pathol. 1994;144(2):321.
- Yee NS, Paek I, Besmer P. Role of kit-ligand in proliferation and suppression of apoptosis in mast cells: basis for radiosensitivity of white spotting and steel mutant mice. J Exp Med. 1994;179(6):1777-87.
- Rodewald HR, Dessing M, Dvorak AM, Galli SJ. Identification of a committed precursor for the mast cell lineage. Sci. 1996;271(5250):818-22.
- Bischoff SC, Dahinden CA. c-kit ligand: A unique potentiator of mediator release by human lung mast cells. J Exp Med. 1992;175(1):237-44.
- Columbo M, Horowitz EM, Botana LM, MacGlashan DW, Bochner BS, Gillis S, Zsebo KM, Galli SJ, Lichtenstein LM. The human recombinant c-kit receptor ligand, rhSCF, induces mediator release from human cutaneous mast cells and enhances IgE-dependent mediator release from both skin mast cells and peripheral blood basophils. J Immunol. 1992;149(2):599-608.
- Meininger CJ, Yano H, Rottapel R, Bernstein A, Zsebo KM, Zetter BR. The c-Kit receptor ligand functions as a mast cell chemoattractant. Blood. 1992;79(4):958-63.
- Besmer P. The kit ligand encoded at the murine Steel locus: a pleiotropic growth and differentiation factor. Curr Opin Cell Biol. 1991;3(6):939-46.
- Yamagata S, Minamishima Y,Morisawa S. Fluorospectrophotometric characterization of nucleic acid-acridine orange complexes II. Interaction of nucleic acids or nucleoproteins and acridine orange. Osaka City Med J. 1972;18(1):85-94.
- Krishnamoorthy N, Oriss TB, Paglia M, Fei M, Yarlagadda M, Vanhaesebroeck B, et al. Activation of c-Kit in dendritic cells regulates T helper cell differentiation and allergic asthma. Nat Med. 2008;14(5):565-73.
- Ray P, Krishnamoorthy N, Oriss TB, Ray A. Signaling of c-kit in dendritic cells influences adaptive immunity. Ann N Y Acad Sci. 2010;1183:104.
- Landreth KS, Kincade PW, Lee G, Harrison DE. B lymphocyte precursors in embryonic and adult W anemic mice. J Immunol. 1984;132(6):2724-9.
- Rico-Vargas SA, Weiskopf B, Nishikawa SL, Osmond DG. c-kit expression by B cell precursors in mouse bone marrow. Stimulation of B cell genesis by in vivo treatment with anti-c-kit antibody. J Immunol. 1994;152(6):2845-52..
- Godfrey DI, Zlotnik A, Suda T. Phenotypic and functional characterization of c-kit expression during intrathymic T cell development. J Immunol. 1992;149(7):2281-5.
- Rodewald HR, Kretzschmar K, Swat W, Takeda S. Intrathymically expressed c-kit ligand (stem cell factor) is a major factor driving expansion of very immature thymocytes in vivo. Immunity. 1995;3(3):313-9.
- Bluman EM, Schnier GS, Avalos BR, Strout MP, Sultan H, Jacobson FW, et al. The c-kit ligand potentiates the allogeneic mixed lymphocyte reaction. Blood. 1996;88(10):3887-93.
- Wang H, Pierce LJ, Spangrude GJ. Distinct roles of IL-7 and stem cell factor in the OP9-DL1 T-cell differentiation culture system. Exp Hematol. 2006;34(12):1730-40.
- Laky K, Lefrancois L, Puddington L. Age-dependent intestinal lymphoproliferative disorder due to stem cell factor receptor deficiency: parameters in small and large intestine. J Immunol. 1997;158(3):1417-27.
- Luo D, Chen H, Searles G, Jimbow K. Coordinated mRNA expression of c-Kit with tyrosinase and TRP-1 in melanin pigmentation of normal and malignant human melanocytes and transient activation of tyrosinase by Kit/SCF-R. Melanoma Res. 1995;5(5):303-9.
- Wehrle‐Haller B. The role of Kit‐ligand in melanocyte development and epidermal homeostasis. Pigment Cell Res. 2003;16(3):287-96.
- Wehrle‐Haller B, Weston JA. Receptor tyrosine kinase‐dependent neural crest migration in response to differentially localized growth factors. BioEssays. 1997;19(4):337-45.
- Weston JA. 6 Sequential segregation and fate of developmentally restricted intermediate cell populations in the neural crest lineage. Curr Top Dev Biol. 1991;25:133-53.
- Botchkareva NV, Khlgatian M, Jack Longley B, Botchkarev VA, Gilchrest BA. SCF/c‐kit signaling is required for cyclic regeneration of the hair pigmentation unit. The FASEB J. 2001;15(3):645-58.
- Randall VA, Jenner TJ, Hibberts NA, De Oliveira IO, Vafaee T. Stem cell factor/c-Kit signalling in normal and androgenetic alopecia hair follicles. J Endocrinol. 2008;197(1):11-24.
- Sharov AA, Li GZ, Palkina TN, Sharova TY, Gilchrest BA, Botchkarev VA. Fas and c-kit are involved in the control of hair follicle melanocyte apoptosis and migration in chemotherapy-induced hair loss. J Invest Dermatol. 2003;120(1):27-35.
- Mintz B, Russell ES. Gene‐induced embryological modifications of primordial germ cells in the mouse. J Exp Zool. 1957;134(2):207-37.
- Manova K, Bachvarova RF. Expression of c-kit encoded at the W locus of mice in developing embryonic germ cells and presumptive melanoblasts. Dev Biol. 1991;146(2):312-24.
- Dolci S, Williams DE, Ernst MK, Resnick JL, Brannan CI, Lock LF, et al. Requirement for mast cell growth factor for primordial germ cell survival in culture. Nat. 1991;352(6338):809-11.
- Godin I, Deed R, Cooke J, Zsebo K, Dexter M, Wylie CC. Effects of the steel gene product on mouse primordial germ cells in culture. Nat. 1991;352(6338):807-9.
- Matsui Y, Toksoz D, Nishikawa S, Nishikawa SI, Williams D, Zsebo K, et al. Effect of Steel factor and leukaemia inhibitory factor on murine primordial germ cells in culture. Nat. 1991;353(6346):750-2.
- Yoshinaga KA, Nishikawa SA, Ogawa MI, Hayashi S, Kunisada T, Fujimoto T, et al. Role of c-kit in mouse spermatogenesis: identification of spermatogonia as a specific site of c-kit expression and function. Dev. 1991;113(2):689-99.
- Packer AI, Besmer P, Bachvarova RF. Kit ligand mediates survival of type A spermatogonia and dividing spermatocytes in postnatal mouse testes. Mol Reprod Dev. 1995;42(3):303-10.
- Marziali G, Lazzaro D, Sorrentino V. Binding of germ cells to mutant SId sertoli cells is defective and is rescued by expression of the transmembrane form of the c-kit ligand. Dev Biol. 1993;157(1):182-90.
- Kissel H, Timokhina I, Hardy MP, Rothschild G, Tajima Y, Soares V, et al. Point mutation in kit receptor tyrosine kinase reveals essential roles for kit signaling in spermatogenesis and oogenesis without affecting other kit responses. The EMBO J. 2000;19(6):1312-26.
- Giebel LB, Spritz RA. Mutation of the KIT (mast/stem cell growth factor receptor) protooncogene in human piebaldism. Prod Natl Acad Sci. 1991;88(19):8696-9.
- Sanders KM, Koh SD, Ward SM. Interstitial cells of Cajal as pacemakers in the gastrointestinal tract. Annu Rev Physiol. 2006;68:307-43.
- Lecoin L, Gabella G, Le Douarin N. Origin of the c-kit-positive interstitial cells in the avian bowel. Dev. 1996;122(3):725-33.
- Sanders KM. Ionic mechanisms of electrical rhythmicity in gastrointestinal smooth muscles. Annu Rev Physiol. 1992;54(1):439-53.
- Rothenberg ME, Nusse Y, Kalisky T, Lee JJ, Dalerba P, Scheeren F, et al. Identification of a cKit+ colonic crypt base secretory cell that supports Lgr5+ stem cells in mice. Gastroenterol. 2012;142(5):1195-205.
- Lee Y, Kumagai Y, Jang MS, Kim JH, Yang BG, Lee EJ, et al. Intestinal Lin− c-Kit+ NKp46− CD4− Population Strongly Produces IL-22 upon IL-1β Stimulation. J Immunol. 2013;190(10):5296-305.
- Wang CH, Verma S, Hsieh IC, Hung A, Cheng TT, Wang SY, et al. Stem cell factor attenuates vascular smooth muscle apoptosis and increases intimal hyperplasia after vascular injury. Arteriosclerosis Thromb Vasc Biol. 2007;27(3):540-7.
- Boesiger J, Tsai M, Maurer M, Yamaguchi M, Brown LF, Claffey KP, et al. Mast cells can secrete vascular permeability factor/vascular endothelial cell growth factor and exhibit enhanced release after immunoglobulin E-dependent upregulation of Fcε receptor I expression. J Exp Med. 1998;188(6):1135-45.
- Pedersen M, Löfstedt T, Sun J, Holmquist-Mengelbier L, Påhlman S, Rönnstrand L. Stem cell factor induces HIF-1α at normoxia in hematopoietic cells. Biochem Biophys Res Commun. 2008;377(1):98-103.
- Lindsey JY, Ganguly K, Brass DM, Li Z, Potts EN, Degan S, et al. c-Kit is essential for alveolar maintenance and protection from emphysema-like disease in mice. Am J Respir Crit Care Med. 2011;183(12):1644-52.
- Ishizawa K, Kubo H, Yamada M, Kobayashi S, Numasaki M, Ueda S, et al. Bone marrow‐derived cells contribute to lung regeneration after elastase‐induced pulmonary emphysema. FEBS lett. 2004;556(1-3):249-52.
- Yamada M, Kubo H, Kobayashi S, Ishizawa K, Numasaki M, Ueda S, et al. Bone marrow-derived progenitor cells are important for lung repair after lipopolysaccharide-induced lung injury. J Immunol. 2004;172(2):1266-72.
- Abe S, Boyer C, Liu X, Wen FQ, Kobayashi T, Fang Q, et al. Cells derived from the circulation contribute to the repair of lung injury. American journal of respiratory and critical care medicine. 2004;170(11):1158-63.
- Ishizawa K, Kubo H, Yamada M, Kobayashi S, Suzuki T, Mizuno S, et al. Hepatocyte growth factor induces angiogenesis in injured lungs through mobilizing endothelial progenitor cells. Biochem Biophys Res Comm. 2004;324(1):276-80.
- Ding L, Dolgachev V, Wu Z, Liu T, Nakashima T, Wu Z, et al. Essential role of stem cell factor–c‐Kit signalling pathway in bleomycin‐induced pulmonary fibrosis. J Pathol. 2013;230(2):205-14.
- Morii E, Hirota S, Kim HM, Mikoshiba K, Nishimune Y, Kitamura Y, et al. Spatial expression of genes encoding c-kit receptors and their ligands in mouse cerebellum as revealed by in situ hybridization. Brain Res Dev Brain Res. 1992;65(1):123-6.
- Orr-Urtreger A, Avivi AA, Zimmer YI, Givol DA, Yarden YO, Lonai PE. Developmental expression of c-kit, a proto-oncogene encoded by the W locus. Development. 1990;109(4):911-23.
- Zhang SC, Fedoroff S. Cellular localization of stem cell factor and c‐kit receptor in the mouse nervous system. J Neurosci Res. 1997;47(1):1-5.
- Jin K, Mao XO, Sun Y, Xie L, Greenberg DA. Stem cell factor stimulates neurogenesis in vitro and in vivo. J Clin Invest. 2002;110(3):311-9.
- S Sun L, Lee J, Fine HA. Neuronally expressed stem cell factor induces neural stem cell migration to areas of brain injury. J Clin Invest. 2004;113(9):1364-74.
- Hirata T, Morii E, Morimoto M, Kasugai T, Tsujimura T, Hirota S, et al. Stem cell factor induces outgrowth of c-kit-positive neurites and supports the survival of c-kit-positive neurons in dorsal root ganglia of mouse embryos. Development. 1993;119(1):49-56.
- Takagi K, Okuda-Ashitaka E, Mabuchi T, Katano T, Ohnishi T, Matsumura S, et al. Involvement of stem cell factor and its receptor tyrosine kinase c-kit in pain regulation. Neurosci. 2008;153(4):1278-88.
- Fletcher CD, Berman JJ, Corless C, Gorstein F, Lasota J, Longley BJ, et al. Diagnosis of gastrointestinal stromal tumors: a consensus approach. Int J Surg Pathol. 2002;10(2):81-9.
- Miettinen M, Furlong M, Sarlomo-Rikala M, Burke A, Sobin LH, Lasota J. Gastrointestinal stromal tumors, intramural leiomyomas, and leiomyosarcomas in the rectum and anus: a clinicopathologic, immunohistochemical, and molecular genetic study of 144 cases. Am J Surg Pathol. 2001;25(9):1121-33.
- Miettinen M, Sarlomo-Rikala M, Lasota J. Gastrointestinal stromal tumors: recent advances in understanding of their biology. Hum Pathol. 1999;30(10):1213-20.
- Miettinen M, Sarlomo-Rikala M, Sobin LH, Lasota J. Esophageal stromal tumors: a clinicopathologic, immunohistochemical, and molecular genetic study of 17 cases and comparison with esophageal leiomyomas and leiomyosarcomas. Am J Surg Pathol. 2000;24(2):211-22.
- Tworek JA, Goldblum JR, Weiss SW, Greenson JK, Appelman HD. Stromal tumors of the abdominal colon: a clinicopathologic study of 20 cases. Am J Surg Pathol. 1999;23(8):937-45.
- Tworek JA, Goldblum JR, Weiss SW, Greenson JK, Appelman HD. Stromal tumors of the anorectum: a clinicopathologic study of 22 cases. Am J Surg Pathol. 1999;23(8):946-54.
- Miettinen M, Sobin LH, Sarlomo-Rikala M. Immunohistochemical spectrum of GISTs at different sites and their differential diagnosis with a reference to CD117 (KIT). Modern Pathol. 2000;13(10):1134-42.
- Wang WL, Conley A, Reynoso D, Nolden L, Lazar AJ, George S, et al. Mechanisms of resistance to imatinib and sunitinib in gastrointestinal stromal tumor. Cancer Chemother Pharmacol. 2011;67(1):15-24.
- Heinrich MC, Rubin BP, Longley BJ, Fletcher JA. Biology and genetic aspects of gastrointestinal stromal tumors: KIT activation and cytogenetic alterations. Hum Pathol. 2002;33(5):484-95.
- Miettinen M, Lasota J. Gastrointestinal stromal tumors-definition, clinical, histological, immunohistochemical, and molecular genetic features and differential diagnosis. Virchows Archiv. 2001;438(1):1-12.
- Franquemont DW. Differentiation and risk assessment of gastrointestinal stromal tumors. Am J Clin Pathol. 1995;103(1):41-7.
- Kemmer K, Corless CL, Fletcher JA, McGreevey L, Haley A, Griffith D, et al. KIT mutations are common in testicular seminomas. AM J Pathol. 2004;164(1):305-13.
- Nakai Y, Nonomura N, Oka D, Shiba M, Arai Y, Nakayama M, et al. KIT (c-kit oncogene product) pathway is constitutively activated in human testicular germ cell tumors. Biochem Biophys Res Commun. 2005;337(1):289-96.
- Richie JP. Somatic Mutations of KIT in Familial Testicular Germ Cell Tumours. J Urol. 2005;173(3):833-4.
- Sakuma Y, Sakurai S, Oguni S, Hironaka M, Salto K. Alterations of the c‐kit gene in testicular germ cell tumors. Cancer Sci. 2003;94(6):486-91.
- Tian Q, Frierson Jr HF, Krystal GW, Moskaluk CA. Activating c-kit gene mutations in human germ cell tumors. Am J Pathol. 1999;154(6):1643-7.
- Went PT, Dirnhofer S, Bundi M, Mirlacher M, Schraml P, Mangialaio S, et al. Prevalence of KIT expression in human tumors. J Clin Oncol. 2004;22(22):4514-22.
- Bokemeyer C, Kuczyk MA, Dunn T, Serth J, Hartmann K, Jonasson J, et al. Expression of stem-cell factor and its receptor c-kit protein in normal testicular tissue and malignant germ-cell tumours. J Cancer Res Clin Oncol. 1996;122(5):301-6.
- MEYTS ER, Skakkebkk NE. Expression of the c‐kit protein product in carcinoma‐in‐situ and invasive testicular germ cell tumours. Int J Aandrol. 1994;17(2):85-92.
- Biermann K, Göke F, Nettersheim D, Eckert D, Zhou H, Kahl P, et al. c‐KIT is frequently mutated in bilateral germ cell tumours and down‐regulated during progression from intratubular germ cell neoplasia to seminoma. J Pathol. 2007;213(3):311-8.
- Looijenga LH, De Leeuw H, Van Oorschot M, Van Gurp RJ, Stoop H, Gillis AJ, et al. Stem cell factor receptor (c-KIT) codon 816 mutations predict development of bilateral testicular germ-cell tumors. Cancer Res. 2003;63(22):7674-8.
- Coffey J, Linger R, Pugh J, Dudakia D, Sokal M, Easton DF, et al. Somatic KIT mutations occur predominantly in seminoma germ cell tumors and are not predictive of bilateral disease: report of 220 tumors and review of literature. Genes, Chromos Cancer. 2008;47(1):34-42.
- Sever M, Jones TD, Roth LM, Karim FW, Zheng W, Michael H, et al. Expression of CD117 (c-kit) receptor in dysgerminoma of the ovary: diagnostic and therapeutic implications. Mod Pathol. 2005;18(11):1411-6.
- Cheng L, Roth LM, Zhang S, Wang M, Morton MJ, Zheng W, et al. KIT gene mutation and amplification in dysgerminoma of the ovary. Cancer. 2011;117(10):2096-103.
- Rottem M, Okada T, Goff JP, Metcalfe DD. Mast cells cultured from the peripheral blood of normal donors and patients with mastocytosis originate from a CD34+/Fc epsilon RI-cell population. Blood. 1994;84(8):2489-96.
- Kirshenbaum AS, Goff JP, Kessler SW, Mican JM, Zsebo KM, Metcalfe DD. Effect of IL-3 and stem cell factor on the appearance of human basophils and mast cells from CD34+ pluripotent progenitor cells. J Immunol. 1992;148(3):772-7.
- Valent P, Spanblochl E, Sperr WR, Sillaber C, Zsebo KM, Agis H, et al. Induction of differentiation of human mast cells from bone marrow and peripheral blood mononuclear cells by recombinant human stem cell factor/kit-ligand in long-term culture. Blood. 1992;80(9):2237-45.
- Valent P, Sillaber C, Bettelheim P. The growth and differentiation of mast cells. Prog Growth Factor Res. 1991;3(1):27-41.
- Sánchez-Muñoz L, Alvarez-Twose I, García-Montero AC, Teodosio C, Jara-Acevedo M, Pedreira CE, et al. Evaluation of the WHO criteria for the classification of patients with mastocytosis. Mod Pathol. 2011;24(9):1157-68.
- Longley BJ, Metcalfe DD, Tharp M, Wang X, Tyrrell L, Lu SZ, et al. Activating and dominant inactivating c-KIT catalytic domain mutations in distinct clinical forms of human mastocytosis. Proc Natl Acad Sci. 1999;96(4):1609-14.
- Longley BJ, Tyrrell L, Lu SZ, Ma YS, Langley K, Ding TG, et al. Somatic c-KIT activating mutation in urticaria pigmentosa and aggressive mastocytosis: establishment of clonality in a human mast cell neoplasm. Nat Genet. 1996;12(3):312-4.
- Nagata H, Worobec AS, Oh CK, Chowdhury BA, Tannenbaum S, Suzuki Y, et al. Identification of a point mutation in the catalytic domain of the protooncogene c-kit in peripheral blood mononuclear cells of patients who have mastocytosis with an associated hematologic disorder. Proc Natl Acad Sci. 1995;92(23):10560-4.
- Bodemer C, Hermine O, Palmérini F, Yang Y, Grandpeix-Guyodo C, Leventhal PS, et al. Pediatric mastocytosis is a clonal disease associated with D816V and other activating c-KIT mutations. J Invest Dermatol. 2010;130(3):804-15.
- Webster JD, Yuzbasiyan-Gurkan V, Kaneene JB, Miller R, Resau JH, Kiupel M. The role of c-KIT in tumorigenesis: evaluation in canine cutaneous mast cell tumors. Neoplasia. 2006;8(2):104-11.
- Chui X, Egami H, Yamashita J, Kurizaki T, Ohmachi H, Yamamoto S, Ogawa M. Immunohistochemical expression of the c-kit proto-oncogene product in human malignant and non-malignant breast tissues. Br J Cancer. 1996;73(10):1233-6.
- Natali PG, Nicotra MR, Sures I, Mottolese M, Botti C, Ullrich A. Breast cancer is associated with loss of the c‐kit oncogene product. Int J Cancer. 1992;52(5):713-7.
- Nielsen TO, Hsu FD, Jensen K, Cheang M, Karaca G, Hu Z, Hernandez-Boussard T, Livasy C, Cowan D, Dressler L, Akslen LA. Immunohistochemical and clinical characterization of the basal-like subtype of invasive breast carcinoma. Clin Cancer Res. 2004;10(16):5367-74.
- Simon R, Panussis S, Maurer R, Spichtin H, Glatz K, Tapia C, et al. KIT (CD117)-positive breast cancers are infrequent and lack KIT gene mutations. Clin Cancer Res. 2004;10(1):178-83.
- Tsutsui S, Yasuda K, Suzuki K, Takeuchi H, Nishizaki T, Higashi H, et al. A loss of c-kit expression is associated with an advanced stage and poor prognosis in breast cancer. Br J Cancer. 2006;94(12):1874-8.
- Tsuura Y, Hiraki H, Watanabe K, Suzuki T, Igarashi S, Shimamura K, et al. Preferential localization of c-kit product in tissue mast cells, basal cells of skin, epithelial cells of breast, small cell lung carcinoma and seminoma/dysgerminoma in human: immunohistochemical study on formalin-fixed, paraffin-embedded tissues. Virchows Archiv. 1994;424(2):135-41.
- Sawyer EJ, Poulsom R, Hunt FT, Jeffery R, Elia G, Ellis IO, Ellis P, Tomlinson IP, Hanby AM. Malignant phyllodes tumours show stromal overexpression of c‐myc and c‐kit. J Pathol. 2003;200(1):59-64.
- Wehrle-Haller B, Weston JA. Soluble and cell-bound forms of steel factor activity play distinct roles in melanocyte precursor dispersal and survival on the lateral neural crest migration pathway. Development. 1995;121(3):731-42.
- Alexeev V, Yoon K. Distinctive role of the cKit receptor tyrosine kinase signaling in mammalian melanocytes. J Invest Dermatol. 2006;126(5):1102-10.
- Huang S, Jean D, Luca M, Tainsky MA, Bar‐Eli M. Loss of AP‐2 results in downregulation of c‐KIT and enhancement of melanoma tumorigenicity and metastasis. The EMBO J. 1998;17(15):4358-69.
- Huang S, Luca M, Gutman M, McConkey DJ, Langley KE, Lyman SD, et al. Enforced c-KIT expression renders highly metastatic human melanoma cells susceptible to stem cell factor-induced apoptosis and inhibits their tumorigenic and metastatic potential. Oncogene. 1996;13(11):2339-47.
- Lassam N, Bickford S. Loss of c-kit expression in cultured melanoma cells. Oncogene. 1992;7(1):51-6.
- Natali PG, Nicotra MR, Winkler AB, Cavaliere R, Bigotti A, Ullrich A. Progression of human cutaneous melanoma is associated with loss of expression of c‐kit proto‐oncogene receptor. Int J Cancer. 1992;52(2):197-201.
- Padua RA, Barrass N, Currie GA. A novel transforming gene in a human malignant melanoma cell line. Nature. 1984;311(5987):671-3.
- Davies H. Mutations of the BRAF gene in human cancer. Nature. 2002;417(6892):949-54.
- Curtin JA, Busam K, Pinkel D, Bastian BC. Somatic activation of KIT in distinct subtypes of melanoma. J Clin Oncol. 2006;24(26):4340-6.
- Rivera RS, Nagatsuka H, Gunduz M, Cengiz B, Gunduz E, Siar CH, et al. C-kit protein expression correlated with activating mutations in KIT gene in oral mucosal melanoma. Virchows Archiv. 2008;452(1):27-32.
- Antonescu CR, Busam KJ, Francone TD, Wong GC, Guo T, Agaram NP, et al. L576P KIT mutation in anal melanomas correlates with KIT protein expression and is sensitive to specific kinase inhibition. Int J Cancer. 2007;121(2):257-64.
- Beadling C, Jacobson-Dunlop E, Hodi FS, Le C, Warrick A, Patterson J, et al. KIT gene mutations and copy number in melanoma subtypes. Clin Cancer Res. 2008;14(21):6821-8.
- Willmore-Payne C, Holden JA, Hirschowitz S, Layfield LJ. BRAF and c-kit gene copy number in mutation-positive malignant melanoma. Hum Pathol. 2006;37(5):520-7.
- Ashida A, Takata M, Murata H, Kido K, Saida T. Pathological activation of KIT in metastatic tumors of acral and mucosal melanomas. Int J Cancer. 2009;124(4):862-8.
- Simon GR, Wagner H. Small cell lung cancer. Chest. 2003;123(1):259S-71S.
- Micke P, Basrai M, Faldum A, Bittinger F, Rönnstrand L, Blaukat A, et al. Characterization of c-kit expression in small cell lung cancer: prognostic and therapeutic implications. Clin Cancer Res. 2003;9(1):188-94.
- Rohr UP, Rehfeld N, Pflugfelder L, Geddert H, Müller W, Steidl U, et al. Expression of the tyrosine kinase c‐kit is an independent prognostic factor in patients with small cell lung cancer. Int J Cancer. 2004;111(2):259-63.
- Hibi K, Takahashi T, Sekido Y, Ueda R, Hida T, Ariyoshi Y, et al. Coexpression of the stem cell factor and the c-kit genes in small-cell lung cancer. Oncogene. 1991;6(12):2291-6.
- Rygaard K, Nakamura T, Spang-Thomsen M. Expression of the proto-oncogenes c-met and c-kit and their ligands, hepatocyte growth factor/scatter factor and stem cell factor, in SCLC cell lines and xenografts. Br J Cancer. 1993;67(1):37-46.
- López-Martin A, Ballestín C, Garcia-Carbonero R, Castaño A, Lopez-Ríos F, López-Encuentra A, et al. Prognostic value of KIT expression in small cell lung cancer. Lung Cancer. 2007;56(3):405-13.
- Boldrini L, Ursino S, Gisfredi S, Faviana P, Donati V, Camacci T, et al. Expression and mutational status of c-kit in small-cell lung cancer: prognostic relevance. Clin Cancer Res. 2004;10(12):4101-8.
- Camps C, Sirera R, Bremnes RM, Garde J, Safont MJ, Blasco A, et al. Analysis of c-kit expression in small cell lung cancer: prevalence and prognostic implications. Lung Cancer. 2006;52(3):343-7.
- Kelly LM, Gilliland DG. Genetics of myeloid leukemias. Annu Rev Genomics Hum Genet. 2002;3(1):179-98.
- Ikeda H, Kanakura Y, Tamaki T, Kuriu A, Kitayama H, Ishikawa J, et al. Expression and functional role of the proto-oncogene c-kit in acute myeloblastic leukemia cells. Blood. 1991;78(11):2962-8.
- Boissel N, Leroy H, Brethon B, Philippe N, De Botton S, Auvrignon A, et al. Incidence and prognostic impact of c-Kit, FLT3, and Ras gene mutations in core binding factor acute myeloid leukemia (CBF-AML). Leukemia. 2006;20(6):965-70.
- Goemans BF, Zwaan CM, Miller M, Zimmermann M, Harlow A, Meshinchi S, et al. Mutations in KIT and RAS are frequent events in pediatric core-binding factor acute myeloid leukemia. Leukemia. 2005;19(9):1536-42.
- Corbacioglu S, Kilic M, Westhoff MA, Reinhardt D, Fulda S, Debatin KM. Newly identified c-KIT receptor tyrosine kinase ITD in childhood AML induces ligand-independent growth and is responsive to a synergistic effect of imatinib and rapamycin. Blood. 2006;108(10):3504-13.
- Tyner JW. RNAi screening of the tyrosine kinome identifies therapeutic targets in acute myeloid leukemia. Blood. 2008;111(4):2238-45.
- Callens C, Chevret S, Cayuela JM, Cassinat B, Raffoux E, De Botton S, et al. Prognostic implication of FLT3 and Ras gene mutations in patients with acute promyelocytic leukemia (APL): a retrospective study from the European APL Group. Leukemia. 2005;19(7):1153-60.
- Care RS, Valk PJ, Goodeve AC, Abu‐Duhier FM, Geertsma‐Kleinekoort WM, Wilson GA, et al. Incidence and prognosis of c‐KIT and FLT3 mutations in core binding factor (CBF) acute myeloid leukaemias. Br J Haematol. 2003;121(5):775-7.
- Nanri T. Mutations in the receptor tyrosine kinase pathway are associated with clinical outcome in patients with acute myeloblastic leukemia harboring t(8;21)(q22;q22). Leukemia. 2005;19(8):1361-6.
- Paschka P, Marcucci G, Ruppert AS, Mrózek K, Chen H, Kittles RA, et al. Adverse prognostic significance of KIT mutations in adult acute myeloid leukemia with inv (16) and t (8; 21): a Cancer and Leukemia Group B Study. J Clin Oncol. 2006;24(24):3904-11.
- Schnittger S, Kohl TM, Haferlach T, Kern W, Hiddemann W, Spiekermann K, et al. KIT-D816 mutations in AML1-ETO-positive AML are associated with impaired event-free and overall survival. Blood. 2006;107(5):1791-9.
- Shimada A, Taki T, Tabuchi K, Tawa A, Horibe K, Tsuchida M, et al. KIT mutations, and not FLT3 internal tandem duplication, are strongly associated with a poor prognosis in pediatric acute myeloid leukemia with t (8; 21): a study of the Japanese Childhood AML Cooperative Study Group. Blood. 2006;107(5):1806-9.
- Babaei MA, Kamalidehghan B, Saleem M, Huri HZ, Ahmadipour F. Receptor tyrosine kinase (c-Kit) inhibitors: a potential therapeutic target in cancer cells. Drug Des Devel Ther. 2016;10:2443.
- Galanis A, Levis M. Inhibition of c-Kit by tyrosine kinase inhibitors. Haematologica. 2015;100(3):e77-9.
- Dubreuil P, Letard S, Ciufolini M, Gros L, Humbert M, Castéran N, et al. Masitinib (AB1010), a potent and selective tyrosine kinase inhibitor targeting KIT. PloS One. 2009;4(9):e7258.
- Frost MJ, Ferrao PT, Hughes TP, Ashman LK. Juxtamembrane mutant V560GKit is more sensitive to Imatinib (sti571) compared with wild-type c-kit whereas the kinase domain mutant d816vkit is resistant 1 supported by a grant from the National Health and Medical Research Council of Australia (NHMRC). MF is recipient of an Australian Postgraduate Award. LKA is a NHMRC Principal Research Fellow. Imatinib was provided by Novartis. 1. Mol Cancer Therap. 2002;1(12):1115-24.
- Martinez-Anton A, Gras D, Bourdin A, Dubreuil P, Chanez P. KIT as a therapeutic target for non-oncological diseases. Pharmacol Therap. 2019;197:11-37.
- Von Bubnoff N, Gorantla SH, Kancha RK, Lordick F, Peschel C, Duyster J. The systemic mastocytosis-specific activating cKit mutation D816V can be inhibited by the tyrosine kinase inhibitor AMN107. Leukemia. 2005;19(9):1670-1.
- Schittenhelm MM, Shiraga S, Schroeder A, Corbin AS, Griffith D, Lee FY, et al. Dasatinib (BMS-354825), a dual SRC/ABL kinase inhibitor, inhibits the kinase activity of wild-type, juxtamembrane, and activation loop mutant KIT isoforms associated with human malignancies. Cancer Res. 2006;66(1):473-81.
- Gajiwala KS, Wu JC, Christensen J, Deshmukh GD, Diehl W, DiNitto JP, et al. KIT kinase mutants show unique mechanisms of drug resistance to imatinib and sunitinib in gastrointestinal stromal tumor patients. Proc Natl Acad Sci. 2009;106(5):1542-7.
- Foster BM, Zaidi D, Young TR, Mobley ME, Kerr BA. CD117/c-kit in cancer stem cell-mediated progression and therapeutic resistance. Biomedicines. 2018;6(1):31.
- Lobo NA, Shimono Y, Qian D, Clarke MF. The biology of cancer stem cells. Annu Rev Cell Dev Biol. 2007;23:675-99.
- Kerr BA, Miocinovic R, Smith AK, West XZ, Watts KE, Alzayed AW, et al. CD117+ cells in the circulation are predictive of advanced prostate cancer. Oncotarget. 2015;6(3):1889.
- Harris KS, Shi L, Foster BM, Mobley ME, Elliott PL, Song CJ, et al. CD117/c-kit defines a prostate CSC-like subpopulation driving progression and TKI resistance. Scient Rep. 2021;11(1):1-3.
- Wiesner C, Nabha SM, Bonfil RD, Dos Santos EB, Yamamoto H, Meng H, et al. C-kit and its ligand stem cell factor: potential contribution to prostate cancer bone metastasis. Neoplasia. 2008;10(9):996-1003.
- Stankov K, Popovic S, Mikov M. C-KIT signaling in cancer treatment. Curr Pharma Des. 2014;20(17):2849-80.
- Longley BJ, Reguera MJ, Ma Y. Classes of c-KIT activating mutations: proposed mechanisms of action and implications for disease classification and therapy. Leukemia Res. 2001;25(7):571-6.
- Kosemehmetoglu K, Kaygusuz G, Fritchie K, Aydin O, Yapicier O, Coskun O, et al. Clinical and pathological characteristics of Gastrointestinal Stromal Tumor (GIST) metastatic to bone. Virchows Archiv. 2017;471(1):77-90.
- Štemberger-Papić S, Vrdoljak-Mozetič D, Verša Ostojić D, Rubeša-Mihaljević R, Krištofić I, Brnčić-Fischer A, et al. Expression of CD133 and CD117 in 64 serous ovarian cancer cases. Collegium Antropologicum. 2015;39(3):745-53.
- Burgos-Ojeda D, Rueda BR, Buckanovich RJ. Ovarian cancer stem cell markers: prognostic and therapeutic implications. Cancer Lett. 2012;322(1):1-7.
- Mainetti LE, Zhe X, Diedrich J, Saliganan AD, Cho WJ, Cher ML, et al. Bone‐induced c‐kit expression in prostate cancer: A driver of intraosseous tumor growth. Int J Cancer. 2015;136(1):11-20.
- Hines SJ, Organ C, Kornstein MJ, Krystal GW. Coexpression of the c-kit and stem cell factor genes in breast carcinomas. Cell Growth Differ. 1995;6(6):769-79.
- Li XY, Jiang LJ, Chen L, Ding ML, Guo HZ, Zhang W, et al. RIG-I modulates Src-mediated AKT activation to restrain leukemic stemness. Mol Cell. 2014;53(3):407-19.
- Jiang T, Qiu Y. Interaction between Src and a C-terminal proline-rich motif of Akt is required for Akt activation. J Biol Chem. 2003;278(18):15789-93.
- Tabu K, Kimura T, Sasai K, Wang L, Bizen N, Nishihara H, et al. Analysis of an alternative human CD133 promoter reveals the implication of Ras/ERK pathway in tumor stem-like hallmarks. Mol Cancer. 2010;9(1):1-5.
- A study of the efficacy and safety of imatinib mesylate in patients with unresectable or metastatic gastrointestinal stromal tumors expressing c-kit gene. [Last accessed on: October 16, 2021] https://ClinicalTrials.gov/show/NCT00237185
- A study of imatinib with reinduction chemotherapy using mitoxantrone, etoposide and cytarabine in patients with relapsed/refractory c-kit positive Acute Myeloid Leukemia (AML). [Last accessed on: October 16, 2021] https://ClinicalTrials.gov/show/NCT01126814
- Safety and efficacy study of nilotinib combined with mitoxantrone, etoposide, and high-dose cytarabine induction chemotherapy followed by consolidation for patients with c-kit positive acute myeloid leukemia. [Last accessed on: October 16, 2021] https://ClinicalTrials.gov/show/NCT01222143
- Dasatinib as therapy for Myeloproliferative Disorders (MPDs). [Last accessed on: October 16, 2021] https://ClinicalTrials.gov/show/NCT00255346
- The child trial: hypoplastic left heart syndrome study. [Last accessed on: October 16, 2021] https://ClinicalTrials.gov/show/NCT03406884
- Combination of mesenchymal and c-kit+ cardiac stem cells as regenerative therapy for heart failure. [Last accessed on: October 16, 2021] https://ClinicalTrials.gov/show/NCT02501811
This work is licensed under Attribution-NonCommercial-NoDerivs 2.0 Generic (CC BY-NC-ND 2.0) International License. With this license readers are free to share, copy and redistribute the material in any medium or format as long as the original source is properly cited.
Author Info
Bathri N Vajravelu1*, Tareq Al-Maqtari2
1Department of Physician Assistant Studies, Massachusetts College of Pharmacy and Health Sciences, Boston, MA, USA
2Department of Pharmacology, Sana’a University, Yemen
*Corresponding Author: Bathri N Vajravelu, PhD, MBBS, MPH, Department of Physician Assistant Studies Massachusetts College of Pharmacy and Health Sciences, Boston, MA, USA;
Email: [email protected]
Copyright
Copyright© 2021 by Vajravelu BN, et al. All rights reserved. This is an open access article distributed under the terms of the Creative Commons Attribution License, which permits unrestricted use, distribution, and reproduction in any medium, provided the original author and source are credited.
Citation
Citation: Vajravelu BN, et al. c-Kit – The Novel Receptor: Physiological Roles, Downstream Signaling and Implications in Cancer. Jour Clin Med Res. 2021;2(3) :1-36.
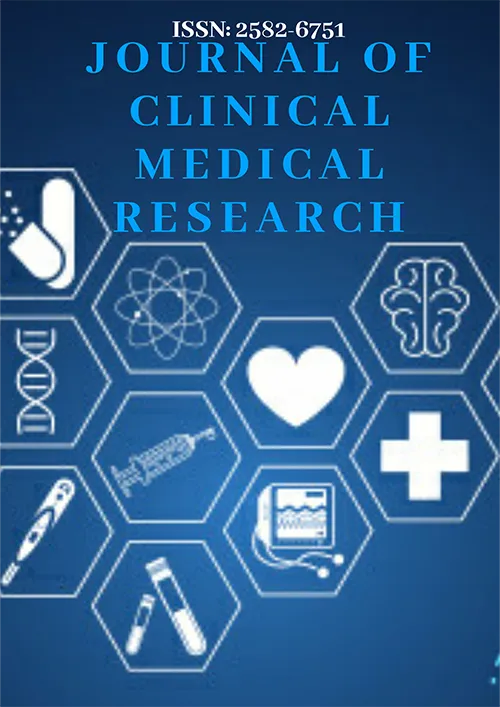