Home » Osmolality Threshold for Sickle Cell Erythrocyte Hemolysis
Research Article | Vol. 1, Issue 3 | Journal of Clinical Medical Research | Open Access |
Osmolality Threshold for Sickle Cell Erythrocyte Hemolysis
Victoria M Richardson1, Kay Woollen, MT1, William A Anong1,2*
1- Department of Clinical Laboratory Science, School of Health Sciences, Winston Salem State University, Winston Salem, North Carolina, USA
2- The Biomedical Research Center, Winston Salem State University, Winston Salem, North Carolina, USA
*Corresponding Author: William A Anong, Department of Clinical Laboratory Science, School of Health Sciences, Winston Salem State University, Winston Salem, North Carolina, USA; Email: [email protected]
Citation: Anong WA, et al. Osmolality Threshold for Sickle Cell Erythrocyte Hemolysis. J Clin Med Res. 2020,1(3):1-9.
Copyright© 2020 by Richardson VM, et al. All rights reserved. This is an open access article distributed under the terms of the Creative Commons Attribution License, which permits unrestricted use, distribution, and reproduction in any medium, provided the original author and source are credited.
Received 24 Aug, 2020 | Accepted 23 Sep, 2020 | Published 02 Oct, 2020 |
Abstract
Background: The importance of fluid intake is well document as metabolic and physiological processes operate effectively in a homeostatic environment. Normal red cells are more resilient to changes in serum osmolality compared to other cells, remaining intact when serum osmolality decreases to as low as 190-mOsM from the 270-290-mOsM reference interval. The membrane’s ability to deform/reform under shear confers the red cell high resistant to changes in serum osmolality. Although normal red cells are resilient, the osmolality threshold for hemolysis of abnormal red cells such as sickle cell blood remains unclear. The primary objective of this study was to determine the osmolality threshold for hemolysis of sickle cell blood vis-a-vis that of normal red cells.
Methods: As described under methods, red cells were prepped and exposed to solution of varying osmolality ranging from 290 to 65-mOsm of sodium chloride. Following incubation, the supernatant and pellets were analyzed for hemoglobin (spectrometry) and Glycophorin A (GPA) content by western blotting techniques.
Results: While the osmolality threshold for normal red cells was 190-mOsM, sickle erythrocytes osmolality threshold for hemolysis was surprisingly 170-mOsM. Both cells ruptured rapidly displaying an S-shaped-like “cooperativity” pattern. Complete (100%) hemolysis occurred at ≤150-mOsM. The hemoglobin retained in pellets decreased to ~50% (normal red cells) and ~20% (sickle red cells) when solution osmolality drops to 65-mOsM.
Conclusion: Sickle cell erythrocytes are more resilient than normal red cells to serum osmolality changes. This finding provides insight into how normal and sickle cell individuals would withstand changes in serum osmolality during dehydration/rehydration states. To alleviate pain, intravenous fluids are routinely administered as adjuvant therapy to slow or reverse the sickling process. Hence, balancing electrolytes and fluid volume during acute pain episodes may significantly benefit African American who are disproportionately afflicted by the sickle cell disease.
Keywords
Erythrocytes; Sickle Cell; Osmolality; Hemolysis; Hemoglobin; Glycophorin A
Introduction
Water drinking is effective in quenching thirst, replacing water loss, maintaining appropriate hydration status and decreasing calorie/energy intake during meals [1,2]. Administered intravenously, isotonic saline (0.85% NaCl) solution is most commonly used in the hospital settings to replenish fluid volume and correct electrolyte imbalances especially in dehydrated elderly patients [3]. Over hydration can lead to fluid overload. In some cases, excessive water creates a hypotonic environment that is deleterious to the proper functioning of many cells and/or incompatible with life [4-6]. In a hypotonic environment, the cells expand and perhaps rupture when the pressure derived from the net influx of water exceeds the membrane’s elastic limit. Hyponatremia is also linked to neurological problems such as loss of appetite, convulsion, obtundation, coma and even death [5,6]. If rapid and severe, the net influx of extracellular fluid creates an edematous brain cells and lung tissues that are incompatible with life [4,6].
Postmortem findings from water intoxication deaths is limited to edema of the brain and lungs. On further examination of specimen taken from the vitreous humor of the eye, serum osmolality was found to be 108-mEq/L (216-mOsM), far lower than the physiological osmolality of 135-145-mEq/L (270-290-mOsM). The red cells functions properly in an isotonic environment of 270-290-mOsM. In a hypotonic environment, the cells swell and burst when the pressure from the next influx of water exceeds the membrane’s elastic limit. We recently reported that normal red cells show resilient to changes in serum osmolality compared to brain and lung cells [7]. Previous evidence indicated that serum osmolality was 216-mOsM on postmortem examinations of individuals who died from water intoxication [5]. Brain and lung cells were severely affected at 216-mOsM. Red cells remain intact at 216-mOsM and only begins to hemolyze when osmolality decreases to 190-mOsM [7]. The ability of red cells to change shape moving through peripheral blood vessels without getting trapped in small capillaries, withstand shear and different osmotic concentrations without bursting is attributed to its membrane composition and the network of membrane bound and cytoskeletal protein interactions.
The flexibility of the normal red cell membrane explains why the osmolality threshold for hemolysis is 190-mOsM and compared to brain and lung cells. Erythrocytes show more resilience to changes in plasma osmolality [7]. However, it is unclear whether such resilient can be associated with abnormal red cells such as sickle red cells or cells with tremendous variability in size and shape. A defect in red cell proteins such as band 3, ankyrin or spectrin leads to compromised membrane stability and increased red cell fragility [8]. Hemoglobin disorders such as the substitution of valine for glutamate in sickle cell disease results in sickled shape red blood cells of various proportions. This genetic disorder is common and leads to periodic episodes of painful crisis when the sickled shape cells traps and occludes blood flow. Exacerbated by decreased fluid volume, the sickling process is managed with medication such as L-glutamine and hydroxyurea and fluid replacements [9]. The inability of the kidney to properly regulate fluid volume in sickle cell disease calls for periodic hydration to slow or stop the sickling process. Fluid replacement increases the plasma volume and decreases the painful episodes by decreasing blood viscosity and indirectly reducing cellular dehydration as well as the intracellular concentration of Hemoglobin S in red cells [10]. However, care in administering fluid is vital to prevent fluid overload and subsequent adverse events such as heart and lung failure, brain edema and death.
On exposure to changes in plasma osmolality, we hypothesized that sickle red cells are likely to be more fragile than normal red cells because of the compromised cytosolic and cytoskeletal protein network. In other words, sickle red cells will rupture at much higher osmolality than the 190-mOsM threshold for hemolysis reported for normal/intact red cells. In this study, we investigated and compared the hemolysis threshold for sickle and normal red cells to changes in solution osmolality. The finding provides insights into how sickle cells patients would respond to changes in serum osmolality during dehydration/hydration states and vaso-occlusive episodes.
Experimental Methods
IRB approval was obtained from the Winston Salem State University’s Office of Sponsored Research. Healthy volunteers provided fresh whole blood in EDTA tube by venipuncture. The sickle cell blood came from the hematology unit of a local hospital laboratory. Both specimens were processed as previously described [7]. Briefly, the cells were washed three times using 0.85% (290-mOsM) isotonic solution, each wash centrifuged at 3000rpm for 3 min. The packed cells were diluted four folds with saline to make approximately a 25% hematocrit suspension. 50-μl of the 25% suspension was added to 2 ml salt solution (~0.5% final hematocrit) of various osmolality ranging from 290 to 65-mOsM sodium chloride (NaCl).
The mixtures were incubated for 30 minutes at room temperature on a gently rocking platform. Post incubation, the samples were centrifuged for 3 min at 3000 rpm to separate cells from supernatant. The absorbance of the supernatant was measured in a spectrophotometer at 540-nm to determine the Hemoglobin (Hb) content. Furthermore, the packed cells were processed and proteins separated by electrophoresis (SDS-Page), transferred unto a nitrocellulose membrane and probed for hemoglobin and Glycophorin A (GPA) contents with hemoglobin (1:1000) and GPA (1:500) antibodies. The blots were developed and Hb and GPA bands quantified using BIORAD chemidoc imaging systems and gel imaging software to determine the proportion Hb/GPA retained in normal and sickle red cells exposed in isotonic and hypotonic solutions [10].
Results
The sickle cell blood obtained from a local hospital laboratory was confirmed by demonstrating the presence of sickled shaped cells in the peripheral smear. As seen in Fig. 1, the modified Wright-Giemsia stain to the right contained many sickled shaped cells in the investigated sample.
Figure 1: Modified Wright-Giemsia stain of peripheral smear from Normal (left) and SCD RBC showing sickle cell RBC (right).
The graph in Fig. 2 depicts an S-shaped curve-like pattern for red cell hemolysis as a function of solution osmolality in both normal and sickle cell red cells. Erythrocytes hemolysed resulting in an increased hemoglobin concentration in the solution as the concentration of sodium chloride (osmolality) decreases. The rate of hemolysis is lower for the sickle cell blood resulting in a right-shift for the sickle cell curve compared to the curve derived from the normal erythrocytes. For the normal and sickle cells blood, 190-mOsM and 170-mOsM, respectively was the minimum threshold osmolality for hemolysis. At 170-mOsM, almost 50% of the normal red cells were lysed. Below the 150-mOsM, both normal and sickled red cells were completely hemolysed resulting in approximately the same amount of hemoglobin in the supernatant.
Figure 2: Graph of normal and Sickle Cell RBC hemolysis as a function of Ionic strength. Red cells (~0.5% hematocrit) was exposed to solution of decreasing salt content and incubated for 30 minutes at room temperature. Following incubation, the Hb content was determined by measuring the absorbance of the supernatant at 540 nm. Each point represent the average to duplicate samples ± error
To corroborate the findings in Fig. 2, the pellets derived from centrifugation were also processed as described under methods and probed for hemoglobin and glycophorin A contents with appropriate antibodies. As indicated in Fig. 3, the hemoglobin content decreased by about 50% and 20% respectively in normal and sickle red cells when solution osmolality drops to 65-mOsM. The sickle cell blot contained approximately the same amount of hemoglobin content at 290 and 170-mOsM. As expected, the GPA retained in the blot was approximately the same in both normal and sickle cell blood irrespective of the solution osmolality.
Figure 3: 20μg sample loaded on 10% SDS gel and probed anti-Hb and anti-GPA antibodies as described under method. Approximately 50% and 80% disappearance of Hb in normal and sickle cell red cells respectively as solution osmolality decreased to 65-mOsM NaCl. GPA retention in membranes was intact and approximately similar in both normal and sickle cells following incubation in solution of different osmolality.
Discussion
The hemolysis curve for sickle cells show a similar “S-shaped” pattern as normal red cells on exposure to decreasing solution osmolality. Surprisingly, we found that sickle erythrocytes showed more resilient to changes in osmolality. The hemolysis threshold for sickle red blood cells of was 170-mOsM, more resistant (less fragile) compared to the 190-mOsM for normal red cell.
The previously established osmolality for normal erythrocyte hemolysis is 190-mOsM and some of the factors that influences red cells hemolysis in a hypotonic solution such as variation in cell size, age of the red cells and hemoglobinopathies were discussed [7]. In this study, the threshold for sickle red cells hemolysis is 170-OsM, indicative of more resistance to changes in osmolality than normal cells. Although the osmolality dependent exposure curve for normal and sickle cell erythrocytes show a similar “S-shaped hemolysis pattern” (Fig. 2), a left shift and decreased Hb content is exhibited in the sickle erythrocytes curve. This is true for the same osmolality up to 150-mOsM, more resilient of sickle cells compared to normal red cells. This agrees with previous report that red cells with normal Hemoglobin (HbAA) were more susceptible than sickle cell Hemoglobin (HbSS) to saline hypotonicity [11,12]. Disorder in beta hemoglobin and perhaps other hemoglobinopathies lead to highly resistant red cells on exposure to varying degrees of hypotonic solutions.
The difference in osmolality threshold for hemolysis between normal and sickle cell blood can be explained by the variability in cell sizes and the number or proportion of sickle-shaped (sickling) red cells in the blood. Erythrocytes have an average diameter of 7-8μm, but sickle red cells show remarkable heterogeneity in density, morphology, and rigidity making the affected cells more rigid and “sticky” compared to normal RBCs [13]. A steeper curve reflects the more uniformity in the sizes of the cells, which allow the cells to reach their osmolality threshold for hemolysis at approximately the same time. The proportion and variants of hemoglobin in sickle cell disease may explain the difference in osmolality threshold for hemolysis between the normal and sickle cell blood. Evidence suggests that sickling in sickle cells blood constitute about 5-50% of the total number of red cells in the peripheral smear [14]. The higher the proportion of sickle and fetal hemoglobin compared to the major Hemoglobin (HbAA), which generally constitutes approximately 97% of the total hemoglobin in normal red cells, the more prominent the right shift or differences between the two specimens [15]. Previous study demonstrated that the severity of sickle cell disease increases with age and the proportion of sickle shaped cells [14]. The sickle-shaped cells are more likely to take a longer time to rehydrate, swell and lyse in a hypotonic environment compared to normal cells. When osmolality decreases to 150-mOsM, both normal and sickle red cells lysed releasing approximately about the same Hb content in solution after 30 minutes of incubation (Fig. 2). For the same hematocrit, sickle cell patients have increased fetal hemoglobin but reduced total hemoglobin compared to normal cells [15-17]. It was however, surprising that we measured approximately the same hemoglobin content in the supernatant at much lower osmolality in both normal and sickle red blood cells. While the total hemoglobin content is low in sickle cell disease, it is likely that the membrane bound fraction decreased, thus favoring an increased proportion of the cytosolic to membrane bound fractions of hemoglobin in the supernatant following hemolysis. Hemoglobin exist as cytosolic and membrane bound fractions [17].
In Fig. 3, the proportion of retained Hb/GPD decreases with decreasing solution osmolality. This decreased is even more significant in sickle red cells compared to normal cells at 65-mOsM solution. We attribute this to, perhaps the compromised structure of the sickled beta subunit of hemoglobin impacted the cytoplasmic to membrane bound ratio. Because hemoglobin subunits exist in both cytoplasmic and membrane fractions, the retained hemoglobin at much lower osmolality are most likely membrane bound fractions. The washed off fractions following complete hemolysis are cytoplasmic, and perhaps constituted mostly the compromised beta-subunit.
The severity of sickle cell disease correlates with the proportion of sickle-shaped cells in the peripheral blood smear. Severe hemolysis and vaso-occlusive crisis are more likely and frequent when the fraction of sickle-shaped cells are high. Perhaps, exploring the osmolality threshold for sickle cell blood as a function of the proportion of sickle-shaped cells is worthwhile. Hydration status during the fluid management/treatment is critical to alleviating the acute pain that sickle cell patients endure during vaso-occlusive episodes [14,18,19].
Conclusion
We have expanded our understanding on the physiological and functional properties of normal/intact and sickle red cells on exposure to solutions of varying osmolality. We have demonstrated that:
- 190-mOsM and 170-mOsM are respectively the osmolality threshold for normal intact and sickle red cells hemolysis
- The proportion of hemoglobin retained in sickle red cells was significantly lower than in intact red cells at much lower osmolality
These finding may aid or provide insights into therapeutic interventions directed at correcting electrolyte imbalances and hydration status for patients with dehydration and/or vaso-occlusive crisis in sickle cell disease. Vaso-occlusive crisis is complex and requires multiple interventions such as the use of hydroxyurea, blood transfusion, gene therapy and fluid replacement in treating/managing patients with sickle cell disease. In a gene therapy study, the proportions of sickled red cells in the patient’s blood decreased significantly when compared to untreated patients with the disease. The drop in sickled red cell proportion improved the oxygen saturation results in treated patient’s blood comparable to that of the heterozygous control. Because the kidneys of sickle cell patients are unable to concentrate urine and regulate bodily fluids properly, regular and appropriate fluid administration is necessary. Fluid replacements helps with rehydration, slow or stop the sickling process, decreases the vascular trappings and alleviate the pain symptoms.
Acknowledgment
Our work is supported by Winston Salem State University’s Grant #211304.
References
- Anderson PD, Mehta NN, Wolfe ML, Hinkle CC, Pruscino L, Comiskey LL, et al. Innate immunity modulates adipokines in humans. J Clin Endocrinol Meta. 2007;92(6):2272-9.
- Davy BM, Dennis EA, Dengo AL, Wilson KL, Davy KP. Water consumption reduces energy intake at a breakfast meal in obese older adults. J Am Dietetic Assoc. 2008;108(7):1236-9.
- Lavizzo-Mourey RJ. Dehydration in the elderly: A short review. J Nat Med Assoc. 1987;79(10):1033.
- Farrell DJ, Bower L. Fatal water intoxication. J Clin Pathol. 2003;56(10):803-4.
- Yamashiro M, Hasegawa H, Matsuda A, Kinoshita M, Matsumura O, Isoda K, et al. A case of water intoxication with prolonged hyponatremia caused by excessive water drinking and secondary SIADH. Case Rep Nephrol Dialysis. 2013;3(2):147-52.
- Kawashima W, Hatake K, Kudo R, Nakanishi M, Tamaki S, Kasuda S, et al. Two autopsy cases of water intoxication. J Forensic Res. 2015;6(271):2.
- Tom-Johnson H, Ariwodola O, Anong, WA. Water intoxication: red cells more resilient to changes in osmolality than brain cells. Int J Rec Sci Res. 2019;10(6):32366-9.
- Anong WA, Weis TL, Low PS. Rate of rupture and reattachment of the band 3-ankyrin bridge on the human erythrocyte membrane. J Biol Chem. 2006;281(31):22360-6.
- Fernandez A, Allbright M. Sickle cell anemia: a succinct look into recently tested disease modifying interventions. J Clin Med Res. 2020;1(2):1-10.
- Okomo U, Meremikwu MM. Fluid replacement therapy for acute episodes of pain in people with sickle cell disease. Cochrane Database of Systematic Reviews. 2017;7(7):CD005406.
- Bio-rad.com. [Last accessed: September 24, 2020] https://www.bio-rad.com/en-us/category/chemidoc-imaging-systems?ID
- Adesina OO, Ilesanmi AO, Banjoko V, Olayanju AO, Akele RY, Akinleye WA, et al. Susceptibility of haemoglobin variants to descending grades of hypotonic saline is inversely related to degree of clinical morbidity. Hemoglobin. 2016;42.
- Li X, Dao M, Lykotrafitis G, Karniadakis GE. Biomechanics and biorheology of red blood cells in sickle cell anemia. J Biomechanics. 2017;50:34-41.
- Alvarez O, Montague NS, Marin M, O’Brien R, Rodriguez MM. Quantification of sickle cells in the peripheral smear as a marker of disease severity. Fetal and Pediatric Pathol. 2015;34(3):149-54.
- Franco RS, Yasin Z, Lohmann JM, Palascak MB, Nemeth TA, Weiner M, et al. The survival characteristics of dense sickle cells. Blood, J Am Soc Hematol. 2000;96(10):3610-7.
- Wahed A, Risin S. Chapter 18: Issues with immunology and serology testing. Accurate results in the clinical laboratory: a guide to error detection and correction 2013. Elsevier. 2013;295-304.
- Sant’Ana PG, Araujo AM, Pimenta CT, Bezerra ML, Borges Junior SP, Martins Neto V, et al. Clinical and laboratory profile of patients with sickle cell anemia. Revista Brasileira De Hematologia E Hemoterapia. 2017;39(1):40-5.
- Kakhniashvili DG, Bulla LA, Goodman SR. The human erythrocyte proteome: analysis by ion trap mass spectrometry. Mol Cellular Proteomics. 2004;3(5):501-9.
- Ribeil JA, Hacein-Bey-Abina S, Payen E, Magnani A, Semeraro M, Magrin E, et al. Gene therapy in a patient with sickle cell disease. New Eng J Med. 2017;376(9):848-55.
This work is licensed under Attribution-NonCommercial-NoDerivs 2.0 Generic (CC BY-NC-ND 2.0) International License. With this license readers are free to share, copy and redistribute the material in any medium or format as long as the original source is properly cited.
Author Info
Victoria M Richardson1, Kay Woollen, MT1, William A Anong1,2*
1- Department of Clinical Laboratory Science, School of Health Sciences, Winston Salem State University, Winston Salem, North Carolina, USA
2- The Biomedical Research Center, Winston Salem State University, Winston Salem, North Carolina, USA
*Corresponding Author: William A Anong, Department of Clinical Laboratory Science, School of Health Sciences, Winston Salem State University, Winston Salem, North Carolina, USA; Email: [email protected]
Copyright
Copyright© 2020 by Richardson VM, et al. All rights reserved. This is an open access article distributed under the terms of the Creative Commons Attribution License, which permits unrestricted use, distribution, and reproduction in any medium, provided the original author and source are credited.
Citation
Citation: Anong WA, et al. Osmolality Threshold for Sickle Cell Erythrocyte Hemolysis. J Clin Med Res. 2020,1(3):1-9.
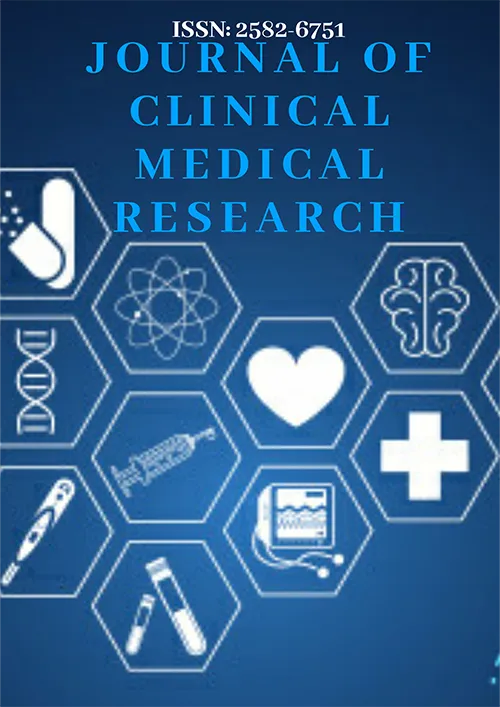