Home » Precision Drugs
Review Article | Vol. 4, Issue 3 | Journal of Clinical Medical Research | Open Access |
Precision Drugs
Karel Petrak1*
1Chief Scientific Officer, NangioTx Inc., Newark, New Jersey, USA
*Correspondence author: Karel Petrak, Chief Scientific Officer, NangioTx Inc., Newark, New Jersey, USA; Email: [email protected]
Citation: Petrak K. Precision Drugs. Jour Clin Med Res. 2023;4(3):1-4.
Copyright© 2023 by Petrak K. All rights reserved. This is an open access article distributed under the terms of the Creative Commons Attribution License, which permits unrestricted use, distribution, and reproduction in any medium, provided the original author and source are credited.
Received 26 Nov, 2023 | Accepted 18 Dec, 2023 | Published 25 Dec, 2023 |
Abstract
The mantra of precision medicine is to prevent and treat diseases by considering individual variability in genes, environment and lifestyle for each person. However, precision drugs to enable precision medicine are not yet available. This article addresses this issue.
Keywords: Precision Medicine; Drugs Targeting; Tumor-Specific Antigens; Gene Therapy
Article Type
Review Article
Introduction
Let us start with a definition: Precision medicine is an approach to prevent and treat diseases that takes into account people’s variations in genes, environment and lifestyle. The US Government-defined parallel activity, the “Precision Medicine Initiative, is to generate the scientific evidence needed to move the concept of precision medicine into clinical practice” [1]. One approach has been “discovering genetic causes of disease and finding new drugs that will target dangerous mutations” [2]. In the first approximation, precision medicine may provide a more accurate diagnosis of diseases such as cancer but may not have the means to offer an improved therapy. Precision drugs that target diseases are needed but are yet to be developed.
There is a parallel between targeted and precision drugs-targeting is to generate pharmacologically effective drug concentration at the site of disease while keeping a very low/minimal drug concentration in the rest of the body, away from the site of disease. Targeted drugs are thus “precision drugs” needed to bring precision medicine into the clinical practice. None of the drugs listed as approved by the FDA in 2022 has been described as a “precision drug” [3]. However, the term “targeting patients” has been used.
Drug Development
Drug Target
By definition, precision drugs need to act directly on the targets of diseases. A part of the precision medicine mission is identifying such targets, particularly genes. Hence, targeted drugs should be able to meet the requirements of precision drugs. However, drugs currently labeled or even approved as “targeted” are insufficiently selective, efficacious and safe [4].
The term “targeting / targeted drug” is used to describe different situations.
A: Drug action is directed to a molecular structure associated with the mechanism of disease but also present on a non-diseased/normal tissue (i.e., Tumor-Associated Antigens (TAAs)
B: Drug action is directed to a molecular structure uniquely associated with the disease site but not with the disease mechanism (i.e., Tumor-Specific Antigens (TSAs)
Employing the above approaches does not generate effective precision drugs. Using approaches that have not worked well enough for decades need to be replaced by a new paradigm [5]. Drug research should pursue the development of highly specific drugs capable of interacting with unique structures associated with newly identified biological mechanisms of disease initiation and progression. The current representative of such drugs are therapeutic antibodies that are not ideal, with their disadvantages stemming from their large size (and hence difficulty accessing disease sites) [6]. A part of the new paradigm is not to rely on the current, conventional drug testing process (i.e., high-enough solubility, broad distribution throughout the body). Drugs having low solubility but exhibiting high selectivity in-vitro (e.g., as determined by binding to specific disease-relevant molecular structures) should not be rejected; instead, it should be recognized that such drugs are ideally suited to be “targeted,” i.e., delivered to and released in their active form at sites of disease. For such new drugs, the appropriate delivery system should be selected from the existing “arsenal” of delivery systems. None of the existing systems may prove to be adequate; hence, new, appropriately “tailored” combinations of drug and delivery systems may need to be invented. Whether the existing “natural” delivery systems (such as extracellular vesicles) could be used effectively remains to be a matter of conjecture [7,8].
Drug Delivery
Developing precision drugs and drug-delivery systems must consider many features associated with the nature of the putative delivery target, the genes. Understanding the involvement of genes and their variations in the human population is essential to understanding disease processes as it forms the foundation for curative therapies, beneficial treatments and preventative measures.
The rare, single-gene disorders, such as cystic fibrosis, phenylketonuria, or hemophilia, represent only the tip of the iceberg of diseases. However, the incidence of rare disorders is approximately 1 in 17 individuals [9]. The ultimate target of precision drugs is our DNA, present within the nucleus as chromosomes, with also a small amount of the mitochondria (the mtDNA or mitochondrial genome). Precision drugs will need to be designed to reach these locations and act on DNA. Apart from identical twins, no two individuals look exactly alike; this is reflected in our DNA. The genomes of two unrelated individuals differ about 0.5% of their DNA (approximately 15 million bp) [10]. Each individual has several hundred known or predicted variants damaging gene functions. Variations between populations must also be considered, such as populations of African ancestry [11].
Gene variations between individuals can make it very difficult to determine which variants are benign and which might be associated with a disease [12]. Many conditions and diseases depend on the genotype at a single locus (or gene), with the outcome following Mendel’s laws of inheritance [13,14]. Over 6,000 phenotypes have been identified for which the molecular basis is known; these phenotypes and the associated genes are collected in the database OMIM [15]. For example, cystic fibrosis is characterized by an autosomal recessive inheritance pattern with over 2,000 different known variants in the CFTR gene/region, with an estimated frequency of 1/2500 to 1/3500 in Caucasians, less common in other ethnic groups. There is still a long way to go in understanding genetic contributions in complex diseases and using such rationally in precision medicine. Further, the environmental context needs to be considered. For example, high-calorie intake and a sedentary lifestyle increase the effect of the genetic risk variants in T2D [16].
Gene therapy has aimed at mitigating recessive and dominant disorders. While numerous gene therapy trials have been conducted, only four therapies have reached approval agencies for clinical use. Again, many hurdles remain to be overcome before significant therapeutic potential using gene therapy and gene correction strategies are realized in clinical use, particularly with regard to safety and efficacy [17]. There are many diseases for which the disease gene targets are already known. The task of precision drug development is clear: focus on 1. generating unique specific structures that would act on these targets and 2. finding ways by which these structures would reach the target in its location [18]. However, no existing drugs, targeted or not, are likely be effective in this context. For example, Herceptin, a breast cancer drug, is designed to treat women whose tumors have a protein called HER2 (Human Epidermal Growth Factor Receptor 2 (HER2)), a transmembrane protein that is a receptor for members of the epidermal growth factor family (EGF family) of extracellular protein ligands [19]. Herceptin binds to HER2 protein, blocking cancer cells from receiving chemical signals to grow. Herceptin used in combination with other treatment interventions, such as chemotherapy, has been reported to result in a longer survival, lower 1-year death rate, longer disease-free progression, high response rate to treatment and a longer response to treatment [20,21]. However, Herceptin is not a good example of what precision drugs need to do. It targets receptors on the surface of breast tumor cells that are only indirectly associated with the disease. Further, it has serious side effects. A significant goal of precision medicine is to implement this strategy broadly in medical care focusing on the right drug at the right dose at the right time for the right patient [22]. The recent development of the pan-genome, the sum of a clade’s genomes in a broader perspective, should advance the development of better diagnostics and treatment of genetic diseases. However, it has been argued that “We’re missing quite a bit of information that can contribute to our knowledge of health disparities and health inequities” [23].
So, How Do We Get the Precision Drugs?
According to the FDA, “Precision care will only be as good as the tests that guide diagnosis and treatment. Next Generation Sequencing (NGS) tests are capable of rapidly identifying or ‘sequencing’ large sections of a person’s genome and are important advances in the clinical applications of precision medicine. Patients, physicians and researchers can use these tests to find genetic variants that help them diagnose, treat and understand more about human disease.” This vague declaration has not advanced precision medicine much. However, the FDA is working to ensure the accuracy of NGS tests so that patients and clinicians can receive accurate and clinically meaningful test results [24]. The vast amount of information generated through NGS poses novel regulatory issues for the FDA. The agency has developed a regulatory approach involving consensus standards, crowd-sourced data and state-of-the-art open-source computing technology to support NGS test development. This approach aims to enable innovation in testing and research and speed access to reliable genetic tests [25]. However, the fact remains that the current FDA drug-product approval process has a 97% failure rate of clinical oncology trials, mainly due to drug efficacy or toxicity goals not being met. The key failure is the lack of understanding of the disease mechanisms and, hence, the lack of identification of disease-relevant molecular targets [26,27].
Conclusion
A new paradigm for the generation of precision drugs needs to be adopted. The first step might be to develop approaches to identify unique molecular structures present in a narrowly defined population of cells to evaluate whether finding such features associated with disease might be realistic. Provided this first step is successful, setting up a fully effective algorithm for identifying such unique structures relevant to diseases will need a major input into Artificial Intelligence from human “brain power.” The input will need to provide guidance on how to get and curate relevant data (published and perhaps even unpublished; regardless, all should be validated), how to test to show that the algorithm is performing as needed and how to improve the approach to answer the initial question that is being asked. All this will require solid guidance from human-level intelligence. It will require the AI to assimilate all the existing data on the topic, all the current assumptions and theories about how disease originates and progresses and how it could be cured. It will need to go beyond extracting information and draw novel conclusions and recommendations on what steps need to be taken to reach the ultimate goal understanding the disease and defining unique molecular structures. It will need to foresee ways of making use of this information. To this end, it must be fully competent in intelligence of the given topic. In other words, it will need to acquire full capabilities in all aspects of human intelligence, such as being able to perceive the real world beyond the information given to it by its programmers. It will need to determine the significance of various parts of the overall task and decide which to focus on. It will need to acquire the manner of processing information in human-thinking terms such as perception, abstraction and memories, apply critical analysis and then remember and recall outcomes as and when needed to synthesize a new, more complex whole. Ultimately, it will need to have the capacity to generate new knowledge and know-how. Will AI be able to speculate and imagine? It will need to. Will it be able to reason? It will have to. Will it be logical where humans often cannot be? Will it handle the “what if” questions, predict and make decisions? All that and more. It would better be. But very likely, intelligent input from humans will be needed all along the way to solve the challenging task at hand.
Conflict of Interest
The author has no conflict of interest to declare.
References
- https://www.webmd.com/cancer/precision-vs-traditional-medicine [Last accessed on: December 18, 2023]
- Fox JL. Obama catapults patient-empowered precision medicine. Nature Biotechnol. 2015;33:325.
- https://www.fda.gov/drugs/new-drugs-fda-cders-new-molecular-entities-and-new-therapeutic-biological-products/new-drug-therapy-approvals-2022#executive-summary. [Last accessed on: December 18, 2023]
- Zhukov NV, Tjulandin SA. Targeted therapy in the treatment of solid tumors: practice contradicts theory. Biochemistry. Biokhimiia. 2008;73(5):605-18.
- Petrak K. A new paradigm for developing effective anti-cancer therapeutics. Canc Therapy Oncol Int J. 2017;4(5):555649.
- Petrak K (2015) Antibodies – The “Magic Bullets” for Drug Delivery? Int J Drug Dev Res. 2015;7:26-8.
- Luan X, Sansanaphongpricha K, Myers I, Chen H, Yuan H. Engineering exosomes as refined biological nanoplatforms for drug delivery. Acta Pharmacol Sin 10. 2017.
- Blencowe H, Moorthie S, Petrou M, Hamamy H, Povey S, Bittles A, et al. Congenital disorders expert group: rare single gene disorders: estimating baseline prevalence and outcomes worldwide. J Community Genet. 2018;9(4):397-406.
- Jackson M, Marks L, May GHW, Wilson JB. The genetic basis of disease. Essays Biochem. 2018;62(5):643-723.
- Agyemang C, Bhopal R, Bruijnzeels M. Negro, black, black African, African Caribbean, African American or what? Labelling African origin populations in the health arena in the 21st J Epidemiol Community Health. 2005;59:1014-8.
- https://bitesizebio.com/technical-channels/genomics-and-epigenetics/ [Last accessed on: December 18, 2023]
- Genomics and Epigenetics. A Better Alternative to Immortalized Cells: Combining CRISPR and iPSCs. 2023.
- Magrinelli F, Balint B, Bhatia KP. Challenges in clinicogenetic correlations: one gene-many phenotypes. Movement Disord Clin Pract. 2021;8(3):299-310.
- Roeder K, Bacanu SA, Sonpar V, Zhang X, Devlin B. Analysis of single-locus tests to detect gene/disease associations. Genet Epidemiol. 2005;28(3):207-19.
- Online mendelian inheritance in man. https://www.omim.org/ [Last accessed on: December 18, 2023]
- Collins FS, Doudna JA, Lander ES, Rotimi CN. Human molecular genetics and genomics important advances and exciting possibilities. N Engl J Med. 2021;384:1-4.
- Cring MR, Sheffield VC. Gene therapy and gene correction: targets, progress and challenges for treating human diseases. Gene Ther. 2022;29:3-12.
- Jackson M, Marks L, May GHW, Wilson JB. The genetic basis of disease. Essays Biochem. 2018;62(5):643-723.
- Herbst RS. Review of epidermal growth factor receptor biology. Int J Radiation Oncol Biol Phys. 2004;59(2 Suppl):21-6.
- Wang J, Xu B. Targeted therapeutic options and future perspectives for HER2-positive breast cancer. Sig Transduct Target Ther. 2019;4:34.
- https://www.healthline.com/health/breast-cancer/her2-positive-survival-rates-statistics#recurrence [Last accessed on: December 18, 2023]
- https://www.nih.gov/about-nih/what-we-do/nih-turning-discovery-into-health/promise-precision-medicine [Last accessed on: December 18, 2023]
- https://www.smithsonianmag.com/smart-news/researchers-reveal-the-pangenome-a-more-diverse-look-at-human-dna-180982164/#:~:text=%E2%80%9CThere%E2%80%99s%20still%20a%20lot%20more%20variation%20that%20needs,tackling%20ethical%20questions%20surrounding%20the%20collection%20of%20DNA [Last accessed on: December 18, 2023]
- FDA advances Precision Medicine Initiative by issuing draft guidance on next generation sequencing-based tests. 2016.
https://www.fda.gov/news-events/press-announcements/fda-advances-precision-medicine-initiative-issuing-draft-guidances-next-generation-sequencing-based [Last accessed on: December 18, 2023]
- https://www.fda.gov/medical-devices/in-vitro-diagnostics/precision-medicine [Last accessed on: December 18, 2023]
- Wong C, Siah K, Lo A. Corrigendum: Estimation of clinical trial success rates and related parameters. Biostatistics. 2018;20(2):273-86.
- Petrak K. A challenge to artificial intelligence: finds molecular structures uniquely and functionally connected to the initiation and progression of Diseases. Acta Scientific Cancer Biol. 2020;4:1-4.
This work is licensed under a Creative Commons Attribution 2.0 International License.
Author Info
Karel Petrak1*
1Chief Scientific Officer, NangioTx Inc., Newark, New Jersey, USA
*Correspondence author: Karel Petrak, Chief Scientific Officer, NangioTx Inc., Newark, New Jersey, USA; Email: [email protected]
Copyright
Copyright© 2023 by Petrak K. All rights reserved. This is an open access article distributed under the terms of the Creative Commons Attribution License, which permits unrestricted use, distribution, and reproduction in any medium, provided the original author and source are credited.
Citation
Citation: Petrak K. Precision Drugs. Jour Clin Med Res. 2023;4(3):1-4.
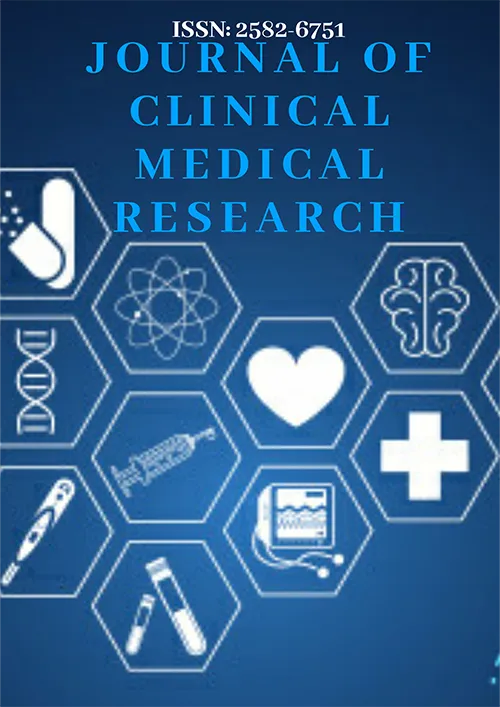