Home » The Periodontal Ligament-A Link between the Cementum and Alveolar Bone
Review Article | Vol. 3, Issue 1 | Journal of Clinical Medical Research | Open Access |
The Periodontal Ligament-A Link between the Cementum and Alveolar Bone
Michel Goldberg1*
1Department of Oral Biology, Faculty of Fundamental and Biomedical Sciences, INSERM UMR-S 1124 Paris Cite University, France
*Corresponding Author: Michel Goldberg, Department of Oral Biology, Faculty of Fundamental and Biomedical Sciences, INSERM UMR-S 1124 Paris Cite University, France; Email: [email protected]
Citation: Goldberg M. The Periodontal Ligament-A Link between the Cementum and Alveolar Bone. Jour Clin Med Res. 2022;3(1):1-18.
Copyright© 2022 by Goldberg M. All rights reserved. This is an open access article distributed under the terms of the Creative Commons Attribution License, which permits unrestricted use, distribution, and reproduction in any medium, provided the original author and source are credited.
Received 24 Jan, 2021 | Accepted 26 Feb, 2022 | Published 05 Mar, 2022 |
Abstract
The Periodontal Ligament (PDL) provides support to the tooth in the bonny socket, in connecting the cementum and alveolar bone. The PDL width vary around 25 mm, including collagens and oxytalan fibers (Sharpey’s fibers). Metalloproteinases play role in the turnover of type I collagen and other matrix proteins. The PDL is implicated in bone remodeling, cells nutrition and sensory events, namely as periodontal mechanoreceptors. The PDL generates and controls tooth eruption and ensures homeostasis between fibroblasts and bone cells. The PDL form, maintain and repair this interface. After dissociation of the cells of the Hertwig’s Epithelial Root Sheath, epithelial rests of Malassez represent a unique source of stem cells population within the periodontal ligament. Extracellular matrix proteins (collagen, fibronectin and Secreted Proteins Acidic and Rich in Cysteine (SPARC), Glycosaminoglycans (GAGs) and Proteoglycans (PGs), Metalloproteinases (MMPs) and Tissue Inhibitors of Metalloproteinases (TIMP- 1 to – 4) are structural components of the PDL. This review explore:
- The attachment function of the PDL (eruption, formative and supportive functions)
- Summarize the implication of stem cells in healing, in the regulation of bone volume, PDL repair and regeneration
- Focus on the contribution of fibroblast-like and pericytes to periodontal inflammation through cytokines and chemokines and the release of molecules implicated in periodontal inflammation
- Sensory events requiring nerve corpuscles (Ruffini-like mechanoreceptors)
- Provide vascular supply and nutrients to the cementum, alveolar bone and to the PDL itself
- Because the cells of the PDL may differentiate into cementoblasts and osteoblasts, this phenotypic availability may contribute to heal the tooth supporting tissues
Keywords
Periodontal Ligament; Anchorage; Radicular Tooth; Alveolar Bone; Cementum; Collagen Fibers; Oxytalan; Matrix Metalloproteinases; Mechanoreceptors; Epithelial Rest of Malassez
Introduction
The periodontal ligament acts as an attachment tissue between bone and tooth, including mainly collagen and oxytalan fibers. This type of ligament is referred to as a synarthrose. Gomphosis is a specialized fibrous joint connecting the cementum to the socket of the maxilla or mandible. It acts as a hydrodynamic damping mechanism during oral food processing. The PDL tissue is anisotropic, heterogeneous, viscoelastic and possesses non-linear elastic properties. The effect of the PDL on strain distribution and/or absorption is restricted locally to the alveolar bone surrounding the teeth and does not affect other regions of the mandible [1-5].
The periodontal ligament is thinner in the middle of the root and slightly wider near the root apex. The “normal” width of the ligament ranges from 0.15 mm to 0.38 mm wide (mean 25 mm), decreasing with age [6]. The functions of the ligament provide support to the teeth, sensory, nutritive and remodeling. In addition to the functions already mentioned, the PDL endures multiaxial loading and therefore is programmed to function under compression, tension, shear and torsion.
The periodontal ligament attaches the tooth to the inner wall of the alveolar bone socket and to cementum. It consists of fibers (crestal, horizontal, oblique and apical), blood vessels (coming from the pulp and gingiva) and from the alveolar bone, playing a critical role as hydrolytic damping and nerves. Alveolar bone and cement are mineralized whereas the periodontal tissue includes the ligament and the lamina propria of gingiva (Fig. 1).
The ligament is composed by fibers, blast and/or clast cells, loose connective tissue, oxytalan fibers and rests of Malassez after dissociation of Herwig’s epithelial root sheath.
Figure 1: Fibers of the periodontal ligament.
Characterization of human periodontal ligament cells in-vitro [7].
Alveolar bone can be divided into two main parts:
- A thin layer of compact bone (the cortex of the alveolus) lining the alveolus proper in which Sharpey’s fibers insert (lamina dura)
- The main alveolar bone surrounding the lamina dura. This alveolar bone remains spongy and porous. This alveolar bone beneath the gingival margin is termed alveolar crest
In addition to the two layers found in all bones, dense compact and cancellous bones form another layer which is called the cribliform plate, lining the alveolar sockets. The lamina dura is normally located around 1 mm below the cemento-enamel junction. Blood vessels and nerves perforate the cribliform plate.
Periodontal ligament comprises five different groups of fibers:
- Alveolar crest; just below the cemento-enamel junction, inserted at the crest of the alveolar bone socket
- Horizontal fibers, below the alveolar crest group. This group originate from the cementum and is directed at right angle to the long axis of the tooth, inserted into the alveolar bone
- Oblique fibers, taking origin from the cementum in an oblique direction, ending into the alveolar bone lining the socket coronally
- Apical fibers are present at the base of the socket. The take origin from the cementum around the apex of the root and they are inserted into the adjacent alveolar bone
- Present only in multi-rooted teeth, interradicular fibers originate from the inter radicular septum of the alveolar bone
- In single rooted teeth, groups of fibers are found forming the:
- Dento-gingival group of fibers
- Alveolo-gingival group
- Circular group
- Dento-periosteal group
The principal fibers ended from part and other in the cementum and in bone (named in such case Sharpey fibers). The ligament has a mean width of 0.25 mm (Fig. 2).
Figure 2: Structure of the periodontium. A: alveolar bone, C: cementum, D: dentin; G: gingiva, P: periodontal ligament, SF: Sharpey’s fibers.
Composition of the Periodontal Ligament Cells
The collagen containing connective tissue: Includes type I, III and V collagens, embedded in the intercellular substance. Collagen fibers have roughly 55 nm in diameter (Fig. 3) [8-11].
Figure 3: Supramolecular assemblies formed by collagens.
Type I collagen is moderate in the periodontal ligament and weaker in bone and cementum. Linked covalently to type I collagen, these fibers contain a high content of cysteine. Type I collagen is found in the periphery of Sharpey’s fibers, forming an attachment to alveolar bone and around nerves and blood vessels.
Type III collagen is formed by 3 identical alpha1 chains with a high content in hydroxyproline and low in hydroxylysine.
Type V collagen is present in small amount in the periodontal ligament.
Type VI collagen is part of the oxytalan fibers system Staining for type VI collagen was strong whereas predentin and dentin were negative [12].
Type XII collagen is present at pressure sites.
Collagen type XIV is expressed in the PDL, forming bundles 5 micrometers in diameter.
Three major groups of integrins are of interest in the PDL: i) I-domain-containing collagen-binding integrins; ii) non-I-Domain integrins linked via ‘bridging’ molecules to collagen and iii) transforming growth factor -β activating integrins [13]. In vertebrates, the integrin family is composed of 18 α-subunits and 8 β-subunits that may assemble into 24 different heterodimers.
Three types of elastic fibers are found in the periodontal ligament: elastin, oxytalan and elaunin.
Oxytalan (immature form of elastin), constitute 3% of the extracellular fibers composition. Oxytalan fibers are naturally elastic. The fibers run both parallel to the root surface and oblique to the root surface. They contain reticulin and elastin. These fibers, 5-2.5 micrometers in diameter, are oval in shape. The functions of oxytalan fibers are tooth support and they contribute to fibroblast migration. Elaunin contains only a small amount of elastin.
The periodontal ligament of rats and microfibrils in the aorta of rats contain vicinal glycol-containing glycoproteins and the PA-TCH-SP method is a useful tool in ultrastructural studies of oxytalan fibers and microfibrils of rats [14]. PA-TCH-SP and Con A staining of carbohydrates is very useful in identifying oxytalan fibers at the ultrastructural level and contain more carbohydrate components than are present in oxytalan fibers rather than in collagen fibrils. PA-TCH-SP and Con A staining of carbohydrates is very useful in identifying oxytalan fibers at the ultrastructural level [15]. Oxytalan fibers consist of bundles of filaments approximately 150 A in diameter with an interfilamentous substance of approximately the same diameter.
Ground Substance
AGs and PGs: Glycoprotein secreted by fibroblasts inhibits mineralization. Hyaluronan: play role in tissue permeability and cell mobility. DS, CS, heparin sulfate; proteodermatan sulphate CS/DS hybrids, decorin and biglycan are components of the ground substance. The components include also fibronectin, tenascin and vitronectin. Glycoproteins and small amount of glycogen are also included in the ground substance composition.
Cells of the Periodontal Ligament
Cells of the periodontal ligament include fibroblasts, defence cells, undifferentiated mesenchymal cells. They gave a PGE2- and isoproterenol-mediated cAMP response, but did not respond in similar fashion to calcitonin or PTH. The periodontal ligament cells produce a bone-associated protein, osteonectin. In addition, osteonectin and bone proteoglycan I (biglycan) were detected in the cells, in-vitro (Table 1).
Fibroblasts display adaptative responses to mechanical forces. Cells are fusiform with many cytoplasmic processes. They are mobile and contractile. TGF-beta promotes tissue matrix formation by stimulating both the synthesis of matrix proteins (collagen, fibronectin and SPARC), Metalloproteinases (MMPs), Proteinase Inhibitors (TIMP-1 to -4) and by decreasing the synthesis of MMPs, but not the 72 kDa-gelatinase (gelatinase A).
Matrix metalloproteinases (MMPs), also known as matrix metallopeptidases or matrixins, are calcium-dependent, zinc-containing endopeptidases. Other family members are adamalysins, serralysins and astacins. The MMPs belong to a larger family of proteases known as the metzincin superfamily.
Gene | Name | Aliases | Location | Description |
MMP1 | Interstitial collagenase | CLG, CLGN | secreted | Substrates include Col I, II, III, VII, VIII, X, gelatin |
MMP2 | Gelatinase-A, 72 kDa gelatinase | secreted | Substrates include Gelatin, Col I, II, III, IV, Vii, X | |
MMP3 | Stromelysin 1 | CHDS6, MMP-3, SL-1, STMY, STMY1, STR1 | secreted | Substrates include Col II, IV, IX, X, XI, gelatin |
MMP7 | Matrilysin, PUMP 1 | MMP-7, MPSL1, PUMP-1 | secreted | membrane associated through binding to cholesterol sulfate in cell membranes, substrates include: fibronectin, laminin, Col IV, gelatin |
MMP8 | Neutrophil collagenase | CLG1, HNC, MMP-8, PMNL-CL | secreted | Substrates include Col I, II, III, VII, VIII, X, aggrecan, gelatin |
MMP9 | Gelatinase-B, 92 kDa gelatinase | CLG4B, GELB, MANDP2, MMP-9 | secreted | Substrates include Gelatin, Col IV, V |
MMP10 | Stromelysin 2 | SL-2, STMY2 | secreted | Substrates include Col IV, laminin, fibronectin, elastin |
MMP11 | Stromelysin 3 | SL-3, ST3, STMY3 | secreted | MMP-11 shows more similarity to the MT-MMPs, is convertase-activatable and is secreted therefore usually associated to convertase-activatable MMPs. Substrates include Col IV, fibronectin, laminin, aggrecan |
MMP12 | Macrophage metalloelastase | HME, ME, MME, MMP-12 | secreted | Substrates include elastin, fibronectin, Col IV |
MMP13 | Collagenase 3 | CLG3, MANDP1, MMP-13 | secreted | Substrates include Col I, II, III, IV, IX, X, XIV, gelatin |
MMP14 | MT1-MMP | MMP-14, MMP-X1, MT-MMP, MT-MMP 1, MT1-MMP, MT1MMP, MTMMP1, WNCHRS | membrane-associated | type-I transmembrane MMP; substrates include gelatin, fibronectin, laminin |
MMP15 | MT2-MMP | MT2-MMP, MTMMP2, SMCP-2, MMP-15, MT2MMP | membrane-associated | type-I transmembrane MMP; substrates include gelatin, fibronectin, laminin |
MMP16 | MT3-MMP | C8orf57, MMP-X2, MT-MMP2, MT-MMP3, MT3-MMP | membrane-associated | type-I transmembrane MMP; substrates include gelatin, fibronectin, laminin |
MMP17 | MT4-MMP | MT4-MMP, MMP-17, MT4MMP, MTMMP4 | membrane-associated | glycosyl phosphatidylinositol-attached; substrates include fibrinogen, fibrin |
MMP18 | Collagenase 4, xcol4, xenopus collagenase | – | No known human orthologue | |
MMP19 | RASI-1, occasionally referred to as stromelysin-4 | MMP18, RASI-1, CODA | – | |
MMP20 | Enamelysin | AI2A2, MMP-20 | secreted | |
MMP21 | X-MMP | MMP-21, HTX7 | secreted | |
MMP23A | CA-MMP | membrane-associated | type-II transmembrane cysteine array | |
MMP23B | – | MIFR, MIFR-1, MMP22, MMP23A | membrane-associated | type-II transmembrane cysteine array |
MMP24 | MT5-MMP | MMP-24, MMP25, MT-MMP 5, MT-MMP5, MT5-MMP, MT5MMP, MTMMP5 | membrane-associated | type-I transmembrane MMP |
MMP25 | MT6-MMP | MMP-25, MMP20, MMP20A, MMPL1, MT-MMP 6, MT-MMP6, MT6-MMP, MT6MMP, MTMMP6 | membrane-associated | glycosyl phosphatidylinositol-attached |
MMP26 | Matrilysin-2, endometase | – | ||
MMP27 | MMP-22, C-MMP | MMP-27 | – | |
MMP28 | Epilysin | EPILYSIN, MM28, MMP-25, MMP-28, MMP25 | secreted | Discovered in 2001 and given its name due to have been discovered in human keratinocytes. Unlike other MMPs this enzyme is constitutivley expressed in many tissues (Highly expressed in testis and at lower levels in lung, heart, brain, colon, intestine, placenta, salivary glands, uterus, skin). A threonine replaces proline in its cysteine switch (PRCGVTD) [14]. |
Table 1: Classification of MMPs and their functions.
Synthetized in the latent form (zymogen), they are secreted as proenzymes and require extracellular activation. They are inhibited by tissue inhibitors of metalloproteinases (TIMPs) (TIMP-1 to TIMP-4) [16-17].
They are grouped into collagenases, gelatinases, stromelysins, et matrilysins. 8 classes structural (5 classes are secreted) and 3 classes are of the membranes type (MT-MMPs).
The MMPs include (Fig. 4)
One peptide signal or pre-domain
One pro-domain, with – proteolytique cleavage, mandatory for the enzyme activation
A catalytic domain with zinc fixation
One hemopexin-like domain
Figure 4: Specific Domains of MMP, ADAM and ADAMTS.
- Fibroclastic cells are involved in collagen degradation. They secrete MMPs, TIMPs, prostaglandins, cytokeratin 19, insulin-like GF and PDGF. BMPs express RANKL (inhibitor of osteoclastic activity). At early passage, PDL cells exhibits contact inhibition, whereas gingival fibroblasts did not. Periodontal ligament cells exhibit significantly distinct characteristics in comparison with gingival fibroblasts. Higher alkaline phosphatase levels are expressed in PDL cells. Differences in aging were noticed. Collagen production was significantly greater in the cells of ligament
- Cementoblasts are forming acellular and cellular cementum
- Osteoblasts: are cuboidal cells loaded by microfilaments. Tight junctions form a transport system in bone (syncytium). Osteoblasts, under the influence of osteotropic hormones (vit D3, PTH and retinoic acid), produce MMPs which appear to remove soft tissue that precludes access of osteoclasts to the mineralized tissue surface [18]
- Osteoclasts/cementoclasts Although there is strong evidence for the involvement of MMPs in the resorption of bone and in the inflammation-mediated destruction of periodontal tissues, the role of MMPs in the remodelling of mature soft connective tissues remains equivocal
- Epithelial rests of Malassez have slow turnover. They contribute to periodontal regeneration [19,20]. The epithelial cell rests of Malassez, rather than being ‘cell rests’ are an important source of stem cells that might play a pivotal role in periodontal regeneration. They also have the capacity to differentiate into a mesenchymal phenotype and thus represent a unique stem cell population within the periodontal ligament (Fig. 5).
Figure 5: Epithelial rests of Malassez.
- Defence cells: such as monocytes/macrophages constitute 4% of the cell population in PDL Mast cells (producing histamine, heparin and factors associated with anaphylaxis). Eosinophils are involved in phagocytosis. Neutrophils and lymphocytes develop in case of inflammation
- Blood vessels: the volume of the lumens vary between 10-30% in the PDL. Fenestrations play role in capillaries
- Nerve: sensory and autonomic (associated with blood vessels) Myelinated sensory have mensurations between 5 mm and up to 15 µm. Unmyelinated both sensory and autonomic (0.5 µm)
- Mechanoreceptors Ruffini -like mechanoreceptor A. They are reactive for cytochrome oxidase activity and acts as neuropeptide Y
- Terminal Schwann cells have both non-specific cholinesterase and acid phosphatase activities
Anatomy
Gingival ligaments: Dento-gingival group, alveolo-gingival group, circular group, dento-periostal group and transseptal fibers.
Mensurations of the PDL: 0.15 to 0.38 mm wide, with a mean value of 25 mm. The thinnest part is located in the middle third of the root. The width of the PDL is reduced over time as the patient ages [7,21]. Ligament composition: water 70%, proteins including the cells associated with innervation, vascularization and the extracellular matrix molecules.
The ligament presented a high density of collagen fibrils grouped in bundles. Oxytalan fibrils were also observed.
Fibroblast-like cells are acting as an immune cell [22]. They are characterized by collagen production and possess some other osteoblastic features. They also produce cytokines and chemokines in response to inflammation promoters.
Innervation
Branches of the Vth cranio-facial nerve arising from the trigeminal nerve through its superior or inferior alveolar branches. The average density of myelinated nerve fibers increased by arriving closer to the apex. The average diameter of myelinated fibers varied between 5.3 μm and 7.8 μm. The innervation is rather close to the alveolar bone. Isolated myelinated axons showed a tendency to group around large blood vessels. Dense innervations by myelinated nerve fibers in close proximity to collagen fibers and alveolar bone, reveals that apical as well as mesial and buccal sites of the human canine are more densely innervated [23].
Two types of neurons were identified: Type I mechanoreceptor containing a single myelinated nerve fibers and unmyelinated nerve fibers and a type II mechanoreceptor. Each receptor had a primary and secondary capsule. Type I receptor received its blood supply by diffusion whereas Type II receptor had a direct blood supply.
Corpuscles of Ruffini are present in the PDL, forming a peripheral innervation. The blood capillaries showed mainly a continuous endothelium, with ending of mechanoreceptors. They are showing little adaptation capacities. They respond to sustained pressure and they are slowly adapting receptors.
Numerous myelinated and non-myelinated nerve fibrils, as well as nerve endings and mechano-receptors were identified in the PDL [24].
Vascular Supply
Vascularization arise from the superior and inferior alveolar arteries. Derived from a series of perforation arteries that pass through the alveolar bone, these vessels occupy interstitial spaces. Many arteriovenous anastomoses occur within the PDL. Lymphatic vessels tend to follow the venous drainage. The abundance of microfilaments and microtubules as well as the presence of intermediary and tight junctions characterize the vascular supply. .
Nexus between the cell processes seemed to indicate that these cells are contractile and motile. The vascular distribution was asymmetric: vascularization was seen to be adjacent to the bone, but not to cementum [25].
Arteries enter the PDL space from the alveolar bone. They are referred to as perforating arteries. From the superior and inferior alveolar (mandibular artery) the apical group of arteries supplying the tooth pulp, alveolar group of arteries, referred to as perforating arteries, dental interseptal artery enter the PDL space and contact anastomosis with gingiva blood vessels. They are referred to as “hydraulic pressure distribution” or “functional regeneration and adaptation”. Mechanical strain transmitted during mastication. Neuropeptides are released and fenestrations allows nutriments to diffuse in the PDL (Fig. 6,7).
The veins drain into the interdental veins or into the periapical plexus.
The lymphatic vessels drain into the regional lymph nodes and finally draining into the thoracic duct.
Figure 6: Vascularization of the dental pulp. Branches rum horizontally and penetrate the alveolar bone to enter the periodontal ligament.
Figure 7: Vascularization of the periodontal ligament.
Proteins Synthetized by the Periodontal Ligament
Extracellular matrix proteins (collagen, fibronectin and Secreted Proteins Acidic and Rich in Cysteine (SPARC), Glycosaminoglycans (GAGs) and Proteoglycans (PGs), Metalloproteinases (MMPs) and Tissue Inhibitors of Metalloproteinases (TIMP- 1 to – 4) are structural components of the PDL [26-27]. The periodontal ligament cells produce osteonectin, a bone-associated protein. Biglycan was detected in-vitro. Periodontal ligament – cells gave a PGE2- and isoproterenol-mediated cAMP response, but did not respond in a similar fashion to calcitonin or PTH.
PDLSCs express common Mesenchymal Stem Cell (MSC) markers and some pericyte markers such as STRO-1, STRO-4, CD29, CD73, CD90 (Thy1), CD106 (VCAM-1) and CD146 (MUC18), but they are negative for the expression of endothelial (CD31), hematopoietic (CD14, CD34, CD45, CD79a) and helper immune antigens (CD11b, CD19, HLA-DR, CD40, CD54, CD80, CD86).
In the PDL, PDLSCs are located in perivascular or paravascular sites. Following culture of the cells for 3 days, high glucose media induced the expression of NANOG, octamer-binding transcription factor 4, (sex determining region Y)-box 2, Cluster of Differentiation 166 (CD166), periostin and β-catenin. Following 14 days in high glucose condition, alkaline phosphatase activity increased. A higher number of calcified nodules were formed on day 28. These results suggest that high glucose induced bone formation by elevating the expression of stem cell markers, particularly CD166 and this induction may be regulated through β catenin [28].
Conclusion
The periodontal ligament constitutes a physical link (Sharpey’s fibers) between the cementum and bone of the alveolar crest. In addition to the PDL functions as support, sensory, nutritive and remodeling, an attachment was induced to the inner bone socket. Epithelial Hertwig epithelial rests may provide stem cells, implicated in the healing and regeneration of the PDL. Tissue repair of the ligament and sensory events are requiring nerve corpuscles (namely Ruffini endings) and vascularization. PDL include collagen and oxytalan fibers, non-phosphorylated molecules (e.g. osteonectin), blood serum derived alpha2-HS-glycoprotein, fibronectin, glycosaminoglycans and proteoglycans, a number of extracellular matrix proteins (including fibronectin and SPARC proteins), metalloproteinases and inhibitors of MMPs. This heterogeneous composition shed lights on the complexity of this structure and to the diversity of functions. It is concluded that PDL has:
- An attachment function to bone and cementum (eruption, formative and supportive function)
- We summarize the implication of stem cells in healing and in the potential roles of epithelial rests of Malassez. The PDL is involved in the regulation of bone volume, PDL repair, tissue homeostasis and regeneration
- Fibroblast-like and pericytes cells of the PDL contribute to periodontal inflammation through cytokines and chemokines. Hence, the cells of the PDL contribute and release molecules implicated in periodontal inflammation
- They are also implicated in sensory events requiring nerve corpuscles (Ruffini-like mechanoreceptors and proprioceptive function) and providing vascular supply and nutrients to the cementum, alveolar bone and to the PDL itself
- Because the cells of the PDL may differentiate into cementoblasts and osteoblasts their phenotypic availability may contribute to the healing of tooth supporting tissues
Conflict of Interest
The author declares no conflict of interest.
References
- Beertsen W, Mcculloch CA, Sodek J. The periodontal ligament: a unique, multifunctional connective tissue. Periodontology. 1997;13:20-40.
- Bosshardt DD, Selvig KA. Dental cementum: the dynamic tissue covering of the root. Periodontology. 1997; 13:41-75.
- Nanci A, Bosshardt DD. Structure of periodontal tissues in health and disease. Periodontology. 2000;40:11-28.
- Berkovitz BK, Holland GR, Moxham B. Oral Anatomy, histology and embryology. 2009.
- Panagiotopoulou O, Kupczik K, Cobb SN. The mechanical function of the periodontal ligament in the macaque mandible: a validation and sensitivity study using finite element analysis. J Anatomy. 2011;218(1): 75-86.
- Mortazavi H, Baharvand M. Review of common conditions associated with periodontal ligament widening. Imaging Science in Dentistry. 2016;46(4):229-37.
- Somerman MJ, Young MF, Foster RA, Moehring JM, Imm G, Sauk JJ. Characteristics of human periodontal ligament cells in-vitro. Archs Oral Biol. 1990;35(3):241-7.
- Berkovitz KB, Moxham BJ, Newman HN. The periodontal ligament in health and disease Mosby-Wolofe. 1995.
- Berkovitz BK. Periodontal Ligament: structural and clinical correlates. Dental Uptake. 2004;31(1):46-50.
- Zeichner-David M. Regeneration of periodontal tissue. Periodontology 2000. 2006;41:196-217.
- Ricard-Blum S. The collagen family. Cold Spring Harb Perspect Biol. 2011;3:a004978.
- Lukinmaa PL, Waltimo J. Immunohistochemical localization of types I, V and VI collagen in human permanent teeth and periodontal ligament. J Dent Res. 1992;71(2):391-7.
- Barczyk M, Bolstad AI, Gullberg D. Role of integrins in the periodontal ligament: organizers and facilitators. Periodontol 2000. 2013;63(1):29-47.
- Takagi M, Parmley RT, Yagasaki H, Toda Y. Ultrastructural cytochemistry of oxytalan fibers in the periodontal ligament and microfibrils in the aorta with the periodic acid-thiocarbohydrazide-silver proteinate method. J Oral Pathol. 1984;13(6):671-8.
- Takagi M, Yagasaki H, Baba T, Baba H. Ultrastructural visualization of carbohydrates in oxytalan fibers in monkey periodontal ligaments. J Histochem Cytochem. 1985;33(10):1007-14.
- Arpino V, Brock M, Gill SE. The role of TIMPs in regulation of extracellular matrix proteolysis. Matrix Biol. 2015;44-46:247-54.
- Behm C, Nemec M, Weissinger F, Rausch MA, Andrukhov O, Jonke E. MMPs and TIMPs expression levels in the periodontal ligament during orthodontic tooth movement: a systematic review of in-vitro and in-vivo Int J Mol Sci. 2021;22(13):6967.
- Sodek J, Overall CM. Matrix metalloproteinases in periodontal remodeling. Matrix Suppl. 1992;1:352-62.
- Rincon JC, Young WG, Bartold PM. The epithelial rests of Malassez- a role in peroiodontal regeneration? J Periodontal Research. 2006;41(4):245-52.
- Xiong J, Gronthos S, Bartold PM. Role of epithelial cell rests of Malassez in the development, maintenance and regeneration of periodontal ligament tissues. Periodontology 2000. 2013;63(1):217-33.
- Somerman MJ, Archer SY, Imm GR, Foster RA. A comparative study of human periodontal ligament cells and gingival fibroblasts in-vitro. J Dent Res. 1988;67(1):66-70.
- Jönsson D, Nebel D, Bratthall G, Nilsson B-O. The human periodontal ligament cell: a fibroblast-like cell acting as an immune cell. J Periodontal Res. 2011;46(2):153-7.
- Huang Y, Corpas LS, Martens W, Jacobs R, Lambrichts I. Histomorphological study of myelinated nerve fibres in the periodontal ligament of human canine. Acta Odontol Scand. 2011;69(5):279-86.
- Frank R, Fellinger E, Steuer P. Ultrastructure of the alveolodental ligament of the rat. J Biol Bucale. 1976; 4(4):295-313.
- Rooker SM, Liu B, Helms JA. The role of Wnt signaling in the biology of the peridontium. Dev Dyn. 2010; 239(1):130-47.
- Decup F, Six N, Palmier B, Buch D, Lasfargues J-J, Salih E, et al. Bone sialoprotein-induced reparative dentinogenesis in the pulp of rat’s molar. Clin Oral Investig. 2000;4(2):110-9.
- Goldberg M. Indirect and Direct Pulp Capping: Reactionary vs Reparative Dentins. JSM Dent. 2021;8(1): 1119.
- Seubbuk S, Sritanaudomchai H, Kasetsuwan J, Surarit R. High glucose promote the osteogenic differentiation capability of human periodontal ligament fibroblasts. Molecular Medicine Reports. 2017;15:2788-94.
This work is licensed under a Creative Commons Attribution 2.0 International License.
Author Info
Michel Goldberg1*
1Department of Oral Biology, Faculty of Fundamental and Biomedical Sciences, INSERM UMR-S 1124 Paris Cite University, France
*Corresponding Author: Michel Goldberg, Department of Oral Biology, Faculty of Fundamental and Biomedical Sciences, INSERM UMR-S 1124 Paris Cite University, France; Email: [email protected]
Copyright
Copyright© 2022 by Goldberg M. All rights reserved. This is an open access article distributed under the terms of the Creative Commons Attribution License, which permits unrestricted use, distribution, and reproduction in any medium, provided the original author and source are credited.
Citation
Citation: Goldberg M. The Periodontal Ligament-A Link between the Cementum and Alveolar Bone. Jour Clin Med Res. 2022;3(1):1-18.
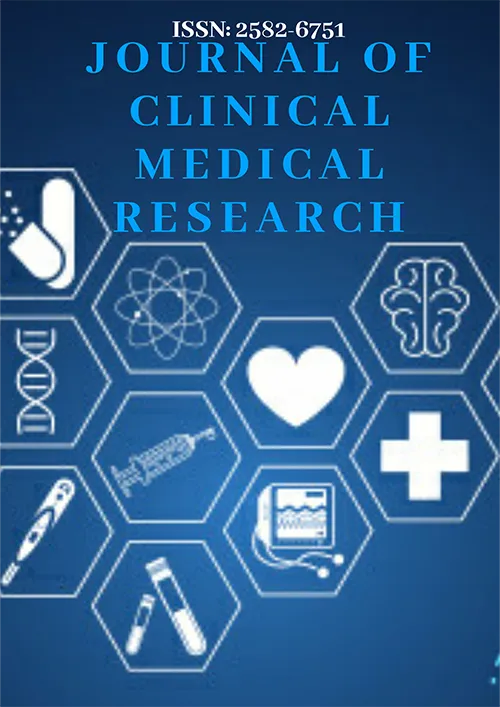